Lead
Chirality, a term derived from the Greek word “cheir,” meaning hand, is a fundamental concept in chemistry and biology that refers to the geometric property of an object not being superimposable on its mirror image. Much like our hands, chiral molecules exist in pairs known as enantiomers, which are mirror images of each other but cannot be aligned perfectly. Despite having the same molecular composition, these enantiomers can behave differently in biological systems due to their three-dimensional structure. Chirality is ubiquitous in nature, influencing the structure and function of biomolecules, and plays a critical role in various industries, particularly pharmaceuticals. The development of methods to separate enantiomers, known as chiral resolution, has become vital, especially when considering the different effects enantiomers can have on biological targets.
1. Introduction to Chirality
Definition and Origin of Chirality
Chirality describes the property of an object or molecule where it cannot be superimposed on its mirror image. The simplest example of this concept is found in human hands. Similarly, in chemistry, molecules can have a chiral center, typically a carbon atom bonded to four distinct groups, giving rise to two distinct forms: enantiomers. These enantiomers are non-superimposable mirror images of each other, much like the left and right hands. Despite being chemically identical, enantiomers can have dramatically different effects when interacting with biological systems due to their three-dimensional shape and orientation.
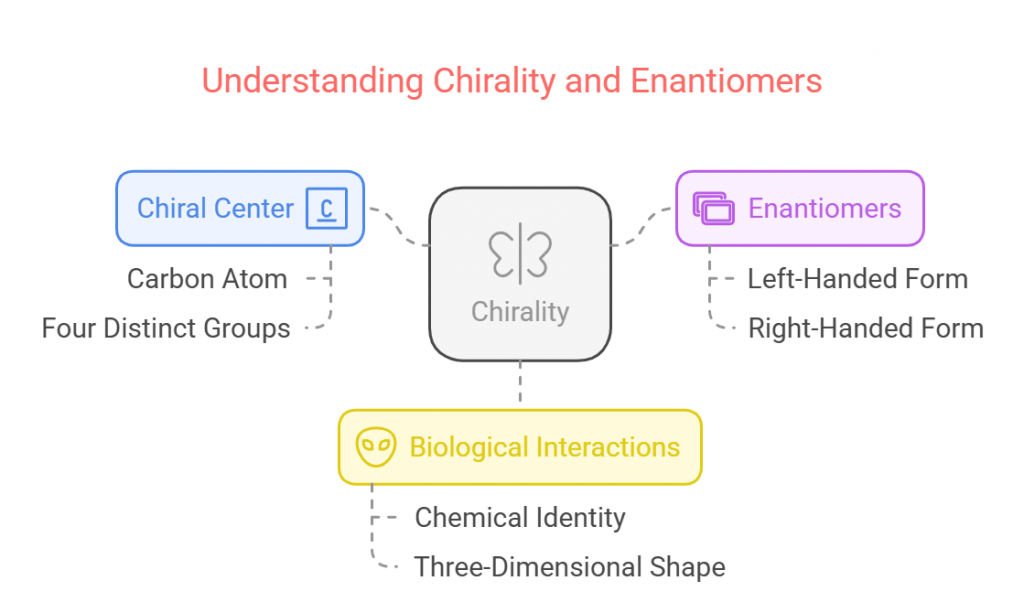

The concept of chirality was first introduced by Louis Pasteur in the mid-19th century when he discovered that certain organic compounds could rotate plane-polarized light in opposite directions. This optical activity was a direct consequence of the molecular asymmetry in these compounds. Pasteur’s work laid the foundation for stereochemistry and the study of chiral compounds, which has since become a crucial area of research in the fields of chemistry, biology, and medicine.
Chirality in Nature and Biology
Chirality is not just a chemical curiosity; it is a fundamental property of biological systems. Most biological molecules, including amino acids and sugars, are chiral, and only one of the enantiomers is naturally occurring. For instance, all naturally occurring amino acids in proteins are in the L-configuration, while the sugars found in nucleic acids are in the D-configuration. This specific handedness of biomolecules means that biological systems, including enzymes and receptors, are highly selective for the chirality of the molecules they interact with (Hangh, 2024).
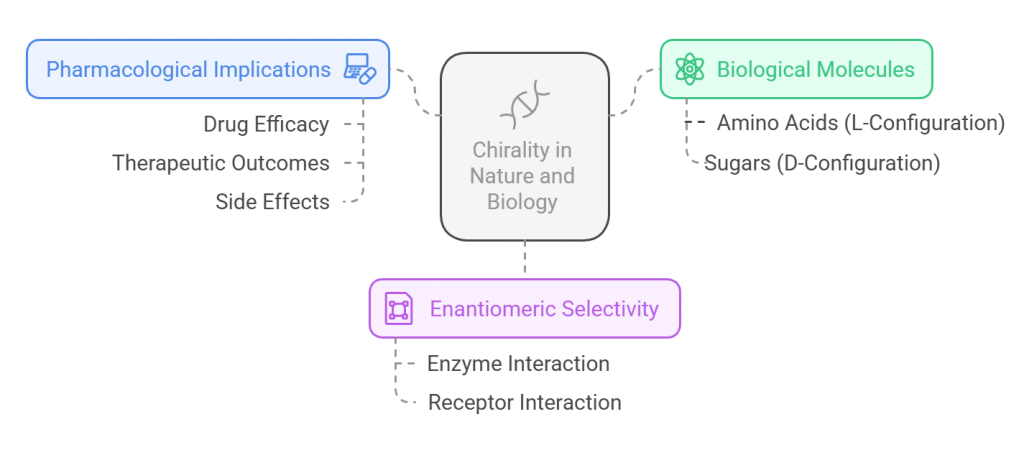
This selectivity has profound implications in pharmacology, where the enantiomeric form of a drug can influence its interaction with biological targets, such as proteins, receptors, and enzymes. For example, the enantiomers of a drug might have different pharmacokinetics, pharmacodynamics, and toxicological profiles, which can lead to significantly different therapeutic outcomes or side effects (Sui, 2023). This underscores the importance of understanding and controlling chirality in drug design and development. For a detailed discussion on he basics of chirality check out the article on <Introduction to Chirality: Understanding the Basics>and <chiral drugs>.
2. Why Chirality Matters in Pharmaceuticals
Drug-Protein Interactions and Enantioselectivity
Enantioselectivity refers to the preferential interaction of one enantiomer over another in biological systems, often dictated by the chiral nature of proteins, enzymes, and receptors. These biological molecules are inherently chiral, meaning that the spatial arrangement of atoms in a drug molecule can significantly influence how it binds to its target.
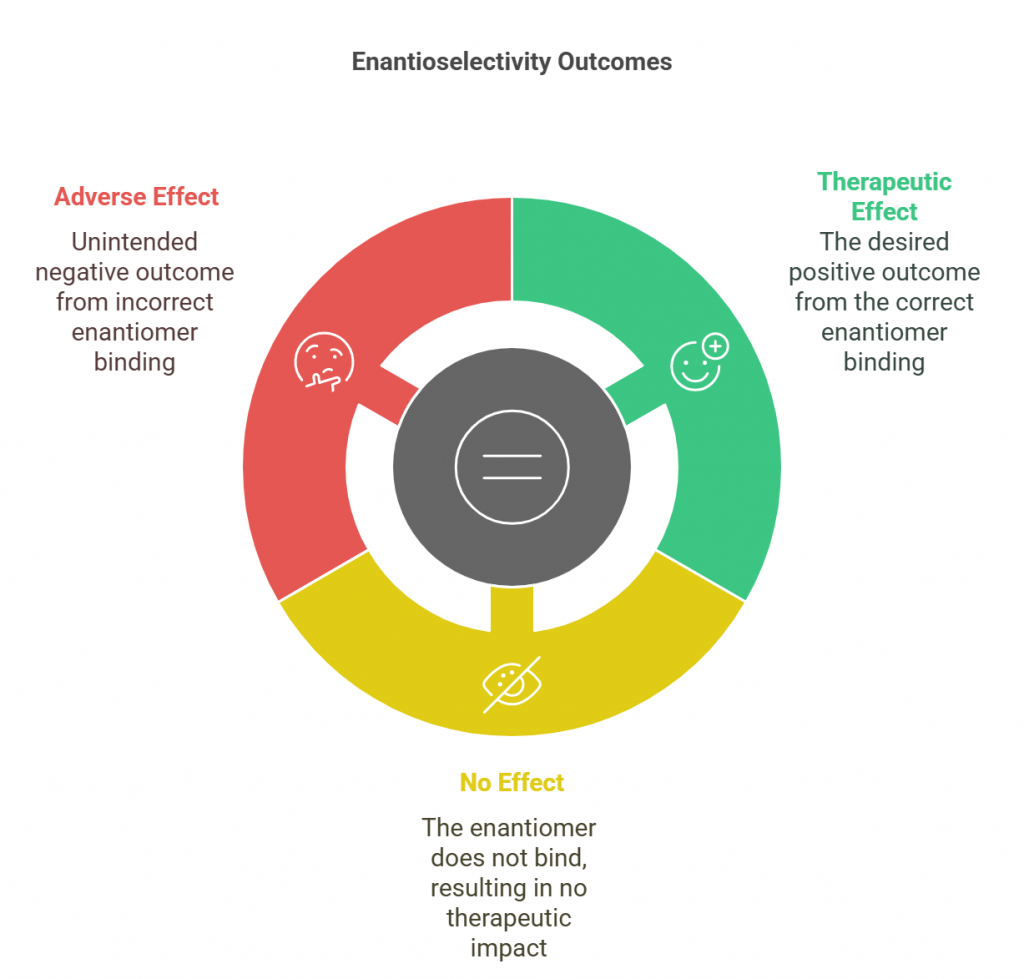
For example, one enantiomer of a drug may fit perfectly into the binding site of an enzyme or receptor, leading to the desired therapeutic effect, while the other may either not fit at all or bind in a way that leads to adverse effects
This specificity is particularly important in drug development, as the difference between the effects of enantiomers can be profound. In some cases, one enantiomer is responsible for the therapeutic effects, while the other may be inactive or even harmful. This has led to the development of single-enantiomer drugs, where only the active enantiomer is used, improving efficacy and reducing potential side effects (Suliman, 2023).
Case Study: The Thalidomide Tragedy and Lessons Learned
One of the most infamous examples of the importance of enantiomer distinction is the case of thalidomide. Thalidomide was introduced in the late 1950s as a sedative and was widely prescribed to pregnant women to alleviate morning sickness. Unfortunately, while the R-enantiomer of thalidomide provided the desired sedative effect, the S-enantiomer caused severe birth defects, leading to one of the worst pharmaceutical disasters in history.
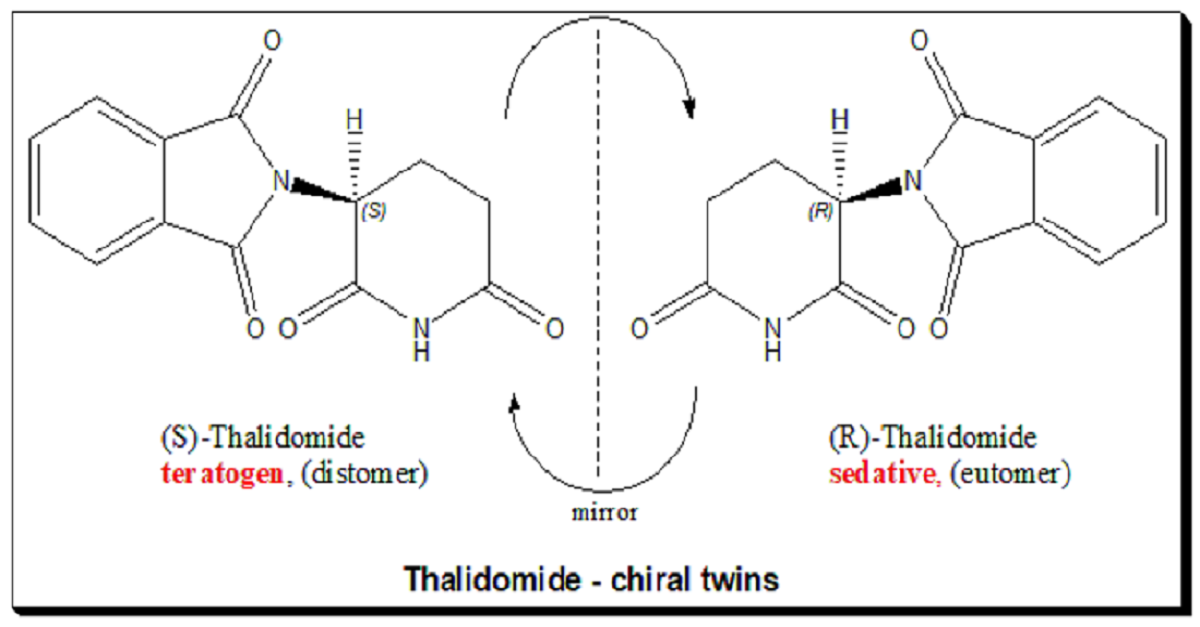
Thalidomide tragedy
This tragedy highlighted the need to understand and control the chirality of pharmaceutical compounds. It led to significant changes in drug development practices, with a greater focus on the enantiomeric composition of drugs and the development of regulatory guidelines that ensure both enantiomers of a chiral drug are thoroughly evaluated for safety and efficacy. The thalidomide disaster remains a critical case study in drug development, emphasizing the importance of chiral resolution.
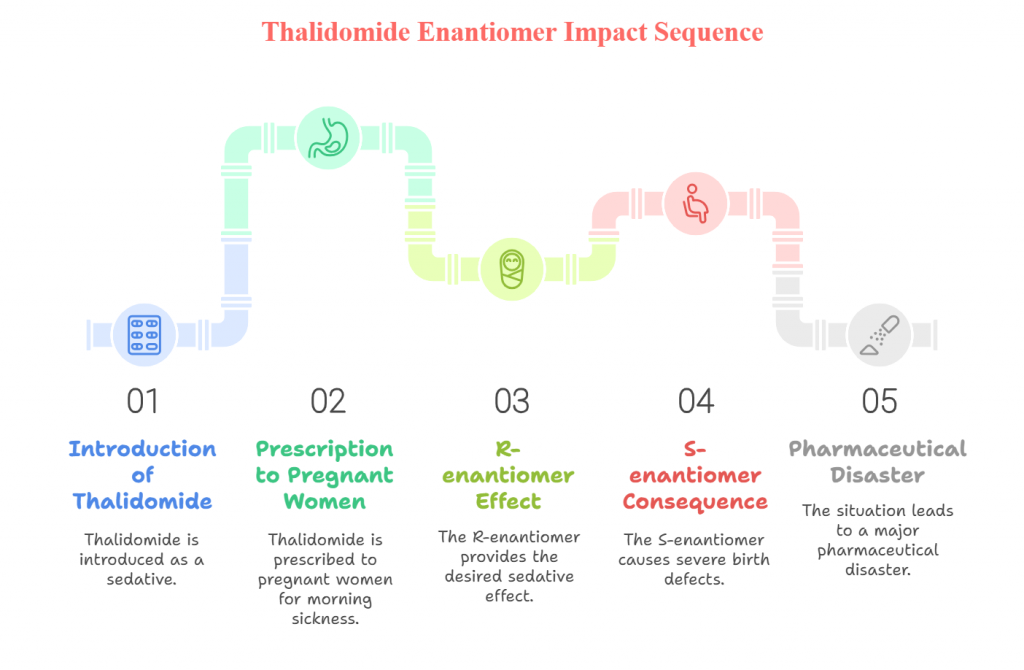
Examples of Drugs with Different Effects from Enantiomers
Beyond thalidomide, many other drugs have enantiomers with different biological effects. For example, ketamine is a well-known anesthetic, but its enantiomers exhibit significantly different pharmacological effects. The S-(+)-enantiomer of ketamine is a potent anesthetic, while the R-(-)-enantiomer has been associated with psychoactive effects and hallucinations. This has led to the development of S-ketamine, a chiral switch, which has fewer side effects compared to the racemic mixture traditionally used.
Similarly, ibuprofen, a common nonsteroidal anti-inflammatory drug (NSAID), exists as two enantiomers. The S-enantiomer (dexibuprofen) is responsible for the drug’s anti-inflammatory effects, while the R-enantiomer is inactive. Interestingly, the body can convert the R-enantiomer into the active S-enantiomer, that is called chiral inversion. For a more detailed discussion on this aspect read the article Chirality in Pharmaceuticals: The Impact of Molecular Handedness on Medicine
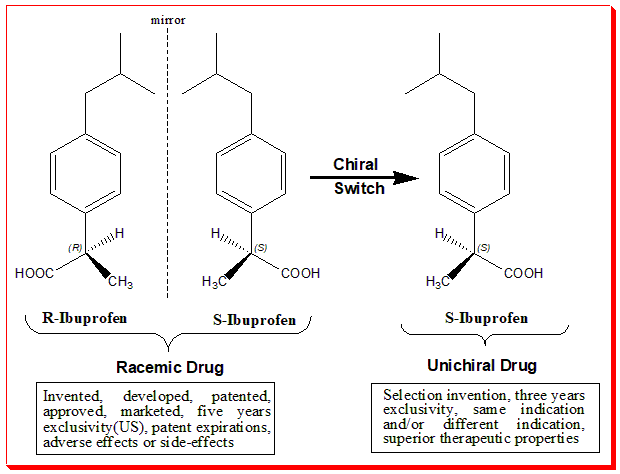
These examples illustrate how the stereochemistry of drugs can influence their therapeutic and adverse effects, further emphasizing the importance of chiral resolution in pharmaceutical development.
3. Historical Context and Key Milestones
Pasteur’s Discovery of Enantiomers
Louis Pasteur’s discovery of molecular chirality in 1848 was a pivotal moment in the field of stereochemistry. He observed that salts of tartaric acid, derived from wine, could rotate polarized light differently depending on their molecular orientation, even though they had the same chemical composition. By manually separating the right-handed and left-handed crystals using a microscope, Pasteur provided the first clear evidence of chirality in molecular structures (Hangh, 2024). This discovery laid the foundation for the study of enantiomers and their separation, which has become a cornerstone of modern stereochemistry. Explore the fascinating journey of chirality from 1809 to 2021, highlighting the key contributions that have shaped its development over the years @ the following article <https://en.wikipedia.org/wiki/Chirality_timeline>.
Development of Chiral Drug Regulations by the FDA
In the 1990s, the U.S. Food and Drug Administration (FDA) recognized the growing importance of chiral drugs and issued guidelines for the development of new stereoisomeric drugs. These guidelines require that drug manufacturers evaluate both enantiomers of a chiral drug, even if only one is intended for therapeutic use. This ensures that the pharmacological, toxicological, and pharmacokinetic properties of each enantiomer are well understood.
These regulations have prompted a shift in drug development, with an increasing focus on single-enantiomer drugs. This shift not only improves the safety and efficacy of medications but also reduces the complexity of drug formulations by eliminating the need to manage the different effects of both enantiomers. The FDA’s guidelines have been instrumental in driving the adoption of chiral resolution techniques in the pharmaceutical industry, ensuring that drugs are both safe and effective for patients.
4. Challenges in Chiral Resolution
The Difficulty of Separating Enantiomers
Separating enantiomers presents a significant challenge due to their identical chemical composition and physical properties. Conventional chemical and physical separation techniques, such as distillation or filtration, are ineffective because enantiomers only differ in their interaction with other chiral substances. As a result, specialized techniques, such as chiral chromatography, crystallization, and membrane-based separations, have been developed to achieve enantiomeric resolution. For more information on the Chiral HPLC separation: strategy and approaches consult the following article <https://chiralpedia.com/blog/chiral-hplc-separation-strategy-and-approaches/>.
These techniques require the use of chiral selectors or agents that can interact differently with each enantiomer, allowing for their separation. However, even with these advanced methods, achieving high purity and efficiency in large-scale chiral separations remains a complex and time-consuming process.
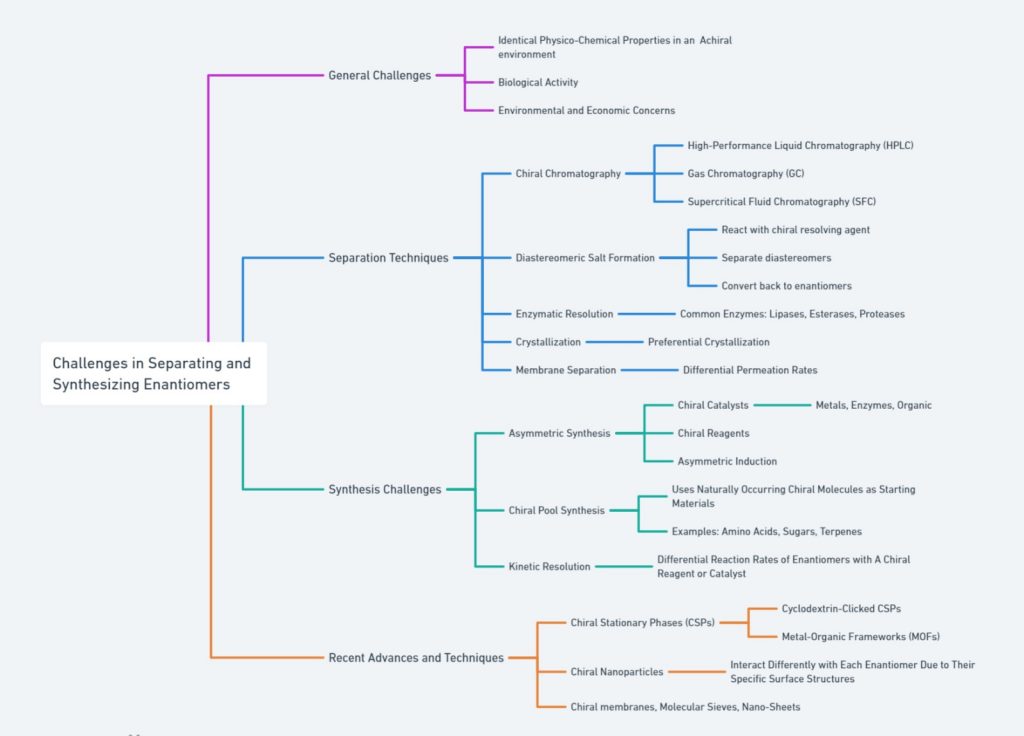
Outlines the challenges and advancements in enantiomer separation and synthesis. It addresses the difficulties posed by identical properties in non-chiral environments, biological activity, and economic factors. Key separation techniques include HPLC, GC, SFC, diastereomeric salt formation, enzymatic resolution, crystallization, and membrane separation. Synthesis challenges involve chiral catalysts, reagents, asymmetric induction, chiral pool synthesis, and kinetic resolution. Recent innovations feature cyclodextrin-clicked CSPs, MOFs, chiral nanoparticles, and advanced chiral membranes. Overall, this comprehensive overview captures the complexities and latest developments in enantiomer separation and synthesis, providing valuable insights for researchers and practitioners in the field.
Why Racemates are Often Undesirable in Drug Development
In many cases, developing racemic mixtures, which contain equal amounts of both enantiomers, is undesirable. This is because one enantiomer may be inactive or, worse, have harmful effects. In drug development, the presence of an inactive or toxic enantiomer can lead to side effects, reduced efficacy, or increased toxicity. As a result, pharmaceutical companies invest significant resources into developing single-enantiomer drugs, which are often more effective and safer for patients (Hangh, 2024).
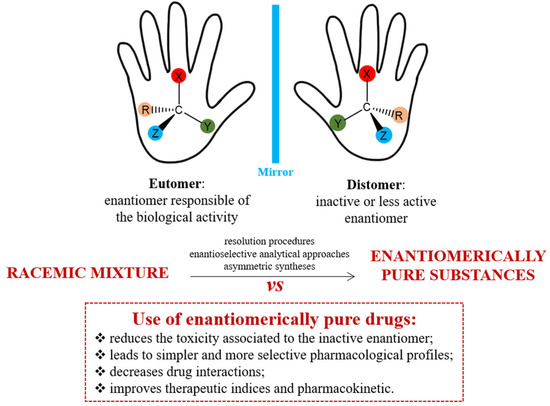
Advantages in the use of enantiopure drugs
Chirality, or “handedness,” is vital in pharmaceutical development, yet many drugs are still sold as racemates. Our bodies, including receptors and enzymes, are chiral and interact specifically with chiral drugs. Therefore, ensuring enantiomeric purity and isolating enantiomers is crucial for drug safety. Notable examples include ibuprofen and thalidomide, which highlight the importance of chirality in preventing toxicity. This remains a key focus for analytical, clinical, and regulatory efforts to improve drug safety.
5. Conclusion
The Critical Importance of Precision in Chiral Resolution
Chiral resolution is not just a technical requirement in drug development – it is a necessity for ensuring the safety and efficacy of pharmaceutical compounds. The ability to distinguish and separate enantiomers allows scientists to design drugs that are not only effective but also free from unnecessary side effects. As the pharmaceutical industry continues to evolve, the precision and efficiency of chiral resolution techniques will remain a key focus, driving innovation and improving patient outcomes. Understanding chirality and its impact on biological systems is essential for the future of medicine, ensuring that new drugs are developed with the highest levels of safety and efficacy.
Further Reading
Hangh, X., 2024. Advancement of Chiral Resolution and Separations: Techniques and Applications. Highlights in Science, Engineering and Technology, 83, pp.305-310.
Pinto, M., 2020. Chiral Separations in Preparative Scale: A Medicinal Chemist’s Viewpoint. Journal of Medicinal Chemistry, 63(14), pp.7889-7899.
Sui, H., 2023. Strategies for Chiral Separation: From Racemate to Enantiomer. Royal Society of Chemistry.
Suliman, A., 2023. Emerging Developments in Separation Techniques and Analysis of Chiral Pharmaceuticals. Molecules, 28(5), pp.1-12.
https://en.wikipedia.org/wiki/Chiral_drugs
https://en.wikipedia.org/wiki/Chiral_switch
https://en.wikipedia.org/wiki/Chiral_inversion
Gal, J. (2013). Molecular Chirality: Language, History, and Significance. In: Schurig, V. (eds) Differentiation of Enantiomers I. Topics in Current Chemistry, vol 340. Springer, Cham. https://doi.org/10.1007/128_2013_435.
https://en.wikipedia.org/wiki/Chirality_timeline