Introduction
Chirality is a fundamental concept in chemistry that plays a crucial role in the structure and function of molecules. Derived from the Greek word for “hand,” chirality refers to the geometric property of a molecule that makes it non-superimposable on its mirror image. This characteristic is significant in various fields, including pharmaceuticals, where the spatial arrangement of atoms within a molecule can drastically affect its biological activity.
Basic Concepts
Stereoisomerism and Chirality
Chirality is a property of a molecule that arises when it cannot be superimposed on its mirror image. Molecules with chiral centers, typically carbon atoms bonded to four different substituents, exist in mirror-image forms. These forms, known as enantiomers and cannot be interconverted without breaking chemical bonds.
Enantiomers and their Behavior
In an achiral environment, enantiomers have identical physical and chemical properties, such as melting points and solubility. But they can exhibit drastically different biological activities, the bio-environment being chiral. This is particularly important in drug development, where one enantiomer may have therapeutic effects, while the other could be inactive or even harmful. The thalidomide tragedy in the 1950s highlighted the importance of enantioselective synthesis, as one enantiomer of the drug caused severe birth defects. Consequently there has been lot of pressure from regulatory authorities and industry across the globe for the production of enantiopure compounds.
Route to Enantiopure Compounds

Different Approaches to obtain Enantiopure Compounds
In response to the exponential research in Medicinal Chemistry concerning the invention, discovery, design, identification and preparation of new biologically active compounds in enantiomerically pure form, aligned to the stricter requirements from regulatory authorities to patent new chiral drug, the development of methods for enantioselective production of chiral compounds as well as evaluation of their enantiomeric purity, proved to be essential. Accordingly, different enantioselective synthetic methodologies were developed in addition to diverse analytical and preparative techniques. Nevertheless, pure enantiomers can be achieved using several methods as illustrated in the image above.
Asymmetric Synthesis
Asymmetric synthesis, also known as enantioselective synthesis, chiral synthesis, is a method used to preferentially produce one enantiomer over another. This technique is essential for creating chiral compounds that are used in a wide range of industries, from medicine to materials science. The ability to control the stereochemistry of molecules is crucial for the development of effective and safe pharmaceutical drugs, as well as for the creation of novel materials with unique properties.
Chirality Timeline

Pioneering Stereochemistry
The study of chirality dates back to the early 19th century with the work of Jean-Baptiste Biot, who discovered the optical activity of certain organic compounds. Later, Louis Pasteur’s separation of tartaric acid crystals into enantiomers marked a significant milestone in chiral chemistry. Pasteur’s work laid the foundation for understanding the importance of molecular handedness. A chronology of significant contributions that have been made in the journey of chirality [1809 to 2021] is available in the article chirality timeline.
Asymmetric synthesis has undergone substantial advancements since its inception. During the mid-20th century, chemists initiated the development of techniques for enantioselective synthesis, which resulted in the discovery of chiral catalysts and ligands. Significant achievements in the field of chemistry include the development of the Sharpless epoxidation, the Corey-Bakshi-Shibata (CBS) reduction, and the Nobel Prize-winning contributions of Knowles, Noyori, and Sharpless in the area of asymmetric catalysis (awarded the Nobel Prize in Chemistry in 2001). Additionally, Benjamin List and David MacMillan were awarded the Nobel Prize in Chemistry in 2021 for their development of a highly accurate method for molecular construction known as organocatalysis.
Catalysis Timeline – Nobel Prize in Chemistry devoted to catalysis
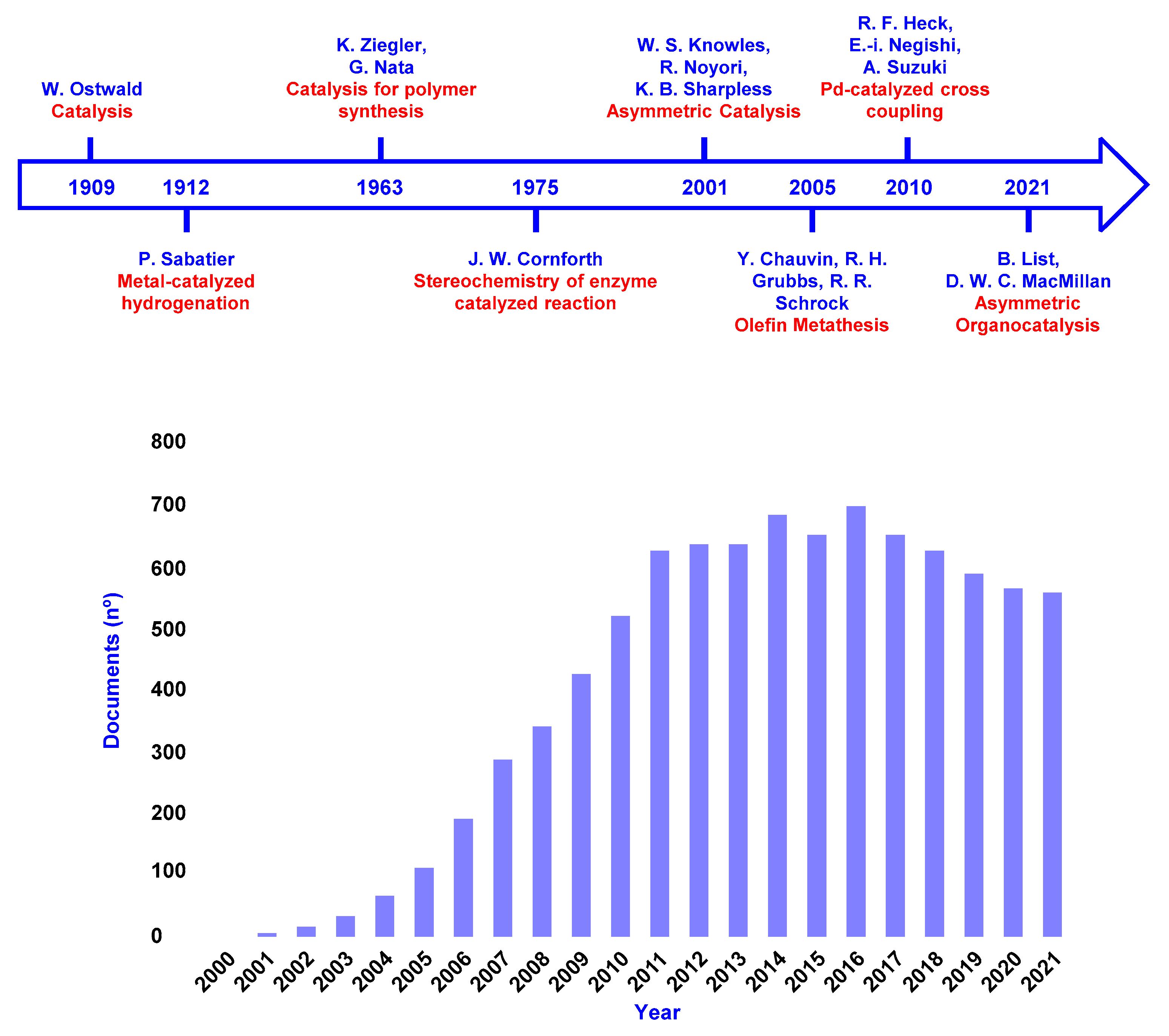
Nobel Prize in Chemistry devoted to catalysis and Number of publications
Historical timeline of the Nobel Prize in Chemistry devoted to catalysis(upper section) and the number of articles containing “organocatalysis” or “organocatalytic” in the title, abstract, or keywords per year (Scopus search, October 2022).(bottom section).
Catalytic Asymmetric Synthesis – What is it?
Catalytic asymmetric synthesis refers to a chemical process that involves the use of a catalyst to produce a desired product with a specific chirality, or handedness. This method allows for the creation of molecules that have a precise three-dimensional structure, which is important in various fields such as pharmaceuticals and materials science.
It is crucial for the industry to have the capability to manufacture products with the highest level of purity. Additionally, it is crucial to possess the capability to produce substantial volumes of a given product. The utilization of catalysts is highly significant due to this rationale. A catalyst is a substance that enhances the reaction rate without undergoing any consumption, and this process or phenomenon is referred to as catalysis.
In recent decades, there has been extensive research focused on developing techniques to synthesize one specific enantiomer instead of its counterpart. Synthesis involves the utilization of substrate molecules to create new molecules (products) through diverse chemical reactions. The Nobel Prize in Chemistry 2001 and 2021 has been awarded to researchers in this field.
The Role of Asymmetric / Chiral Catalysts
Chiral Catalysts are pivotal in asymmetric synthesis as they induce chirality in the products of chemical reactions. These catalysts can be small organic molecules, transition metal complexes, or enzymes, and they function by creating an environment where one enantiomer is formed preferentially. The development of efficient chiral catalysts has been a major focus in the field of organic chemistry.
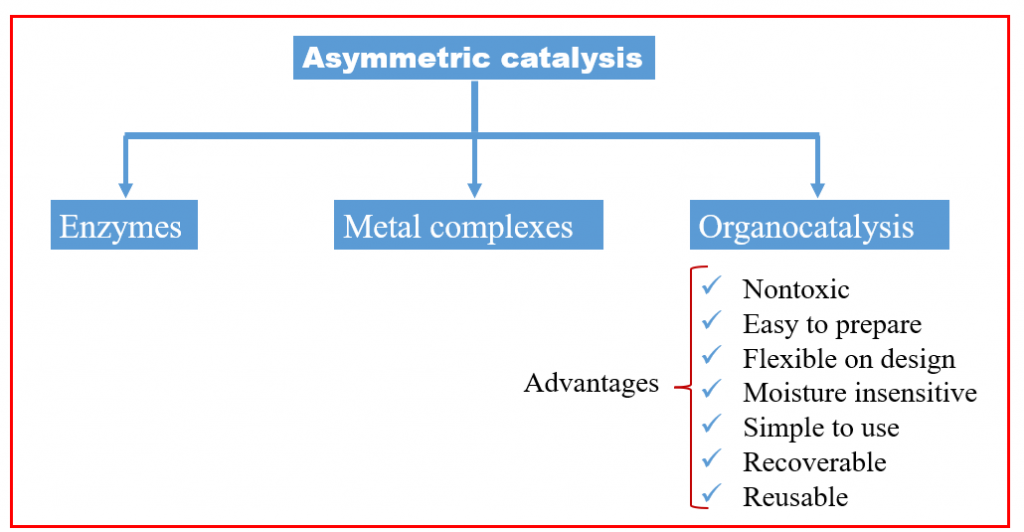
Different Catalytic Approaches
Asymmetric Synthetic Strategies – For Elaboration of enantiopure compounds
Essentially, all catalysts identified prior to the year 2000 can be categorized into two groups: enzymes or metals. Metals are frequently effective catalysts due to their unique capacity to transiently host electrons or transfer them to other molecules during a chemical reaction. This process facilitates the weakening of the connections between the atoms within a molecule, allowing for the breaking of strong bonds and the formation of new ones.
Biocatalysis
Enzymes are nature’s chiral catalysts and have been utilized in synthetic chemistry to achieve enantioselective transformations. Enzyme catalysis offers high specificity and mild reaction conditions. Techniques such as directed evolution have been employed to enhance the enantioselectivity and substrate scope of enzymes.
Biocatalysis has gained significant attention in recent decades because of its potential to enable highly efficient, high-yield, and environmentally friendly processes for producing pharmacologically important compounds. Biocatalysis has proven to be highly effective in synthesizing chiral molecules. Progress in biotechnology and nanotechnology is facilitating the emergence of novel advancements in this domain. There are currently a wide range of strategies available for producing chiral drugs and their intermediate compounds. Enzymes, commonly obtained from biological sources or utilized in whole-cell formats, are eco-friendly tools that offer numerous benefits in catalysis and environmentally conscious reactions. Biocatalytic processes offer improved performance and properties, as well as sustainability, increased efficiency, and cost-effectiveness. This makes it more practical to transition from academic research to industrial application.
Transition-Metal Catalysis
Transition-metal catalysis is one of the most common methods used in asymmetric synthesis. Chiral ligands are often attached to transition metals to create a chiral environment around the metal center, which then facilitates the enantioselective formation of products. Examples include the Rh-catalyzed hydrogenation of alkenes and the Pd-catalyzed allylic substitution reactions.
The Nobel Prize in Chemistry for the year 2001 has been jointly awarded to three scientists: William S. Knowles, previously affiliated with Monsanto Company in St. Louis, Missouri, USA; Ryoji Noyori from Nagoya University in Chikusa, Nagoya, Japan; and K. Barry Sharpless from The Scripps Research Institute in La Jolla, California, USA. The Royal Swedish Academy of Sciences acknowledged their contributions to the advancement of catalytic asymmetric synthesis. Their work has profound implications for academic research, the progress of pharmaceuticals and materials, and has been widely applied in the industrial synthesis of pharmaceuticals and other biologically active compounds.

Enantioselective catalysis. The Chemistry Nobel Prize 2001
The 2001 Nobel Prize for chemistry honours work that allows scientists to make only one version of a molecule that has mirrored forms. The Royal Swedish Academy of Sciences picked out Americans William S Knowles and K Barry Sharpless and Ryoji Noyori of Japan for their pioneering efforts in this field.
Organocatalysis
Organocatalysis involves the use of small organic molecules as catalysts in asymmetric reactions. These catalysts often mimic the function of enzymes and can induce high levels of enantioselectivity. Notable examples include proline-catalyzed aldol reactions and the use of cinchona alkaloids in various transformations.
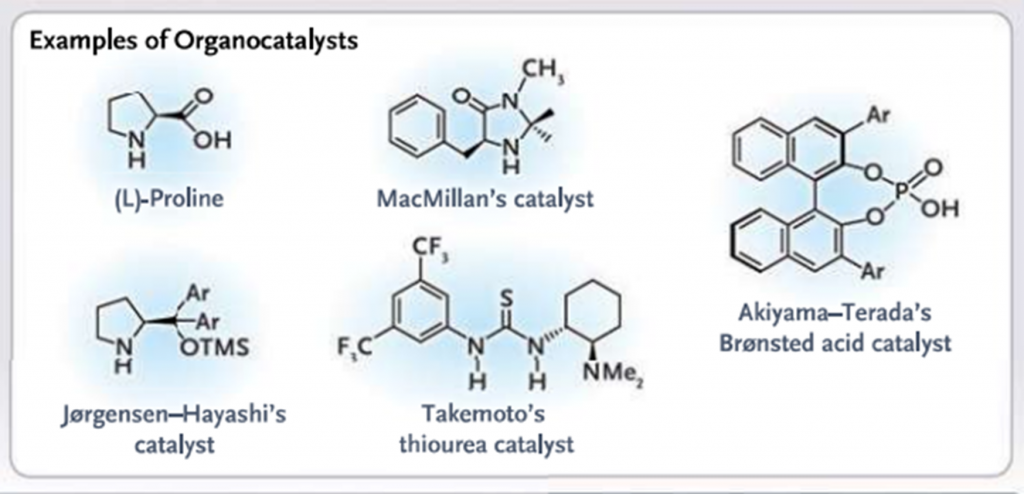
Organocatalysts
Asymmetric Organocatalysis mediated by simple small organic molecules L-Proline, chiral imidazolidinone, thiourea derivatives and Bronsted acids has proved to clean, green and environment friendly tool for the synthesis of enantiomerically compounds
Traditionally, researchers held the belief that catalysts could be classified into two categories: metals and enzymes. However, Benjamin List and David MacMillan received the Nobel Prize in Chemistry in 2021 for their individual contributions to the development of asymmetric organocatalysis in 2000, a third form of catalysis.

Benjamin List and David W.C. MacMillan received the Nobel Prize in Chemistry for deevloping a groundbreaking method/tool to construct molecules.
Their innovation has significantly influenced pharmaceutical research and made chemistry more environmentally friendly. Although consumers may not directly see their work, it plays a vital role in various industries and scientific investigations. List and MacMillan, prominent figures in this domain, have proven that organic catalysts can facilitate a wide range of chemical reactions, allowing scientists to effectively produce novel pharmaceuticals and molecules for solar cells. Thus, organocatalysts offer substantial advantages for humankind.

Asymmetric Synthesis: Three Pillars
The groundbreaking work of Knowles, Noyori, and Sharpless has made asymmetric catalysis one of the leading methods for creating chiral compounds. They primarily used catalysts based on transition metals, which are incredibly versatile and selective in a wide range of chemical reactions. For many years, it was widely believed that metals were the dominant players in this field. However, there are also two other highly effective types of asymmetric catalysts: enzymes (used in biocatalysis) and small organic molecules (used in organocatalysis). Together with metal catalysis, these form what we now call the three pillars of asymmetric catalysis.

Three Pillars of Asymmetric Synthesis
Applications
Pharmaceutical Industry
Chiral drugs represent a significant portion of the pharmaceutical market. Enantioselective synthesis is crucial for producing these drugs in a pure and active form. For instance, the production of (S)-ibuprofen involves the use of chiral catalysts to ensure the desired enantiomer is obtained. A detailed discussion on the asymmetric synthesis of drug molecules, as case studies, will be presented in the upcoming blog on <Asymmetric Synthesis in Industry: From Lab to Market>.
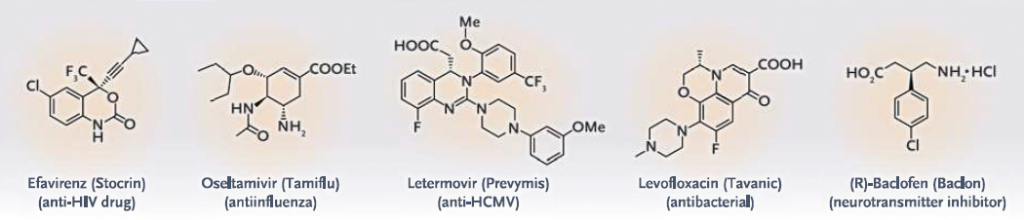
Chiral Drugs synthesized through Organocatalysis
Agrochemicals
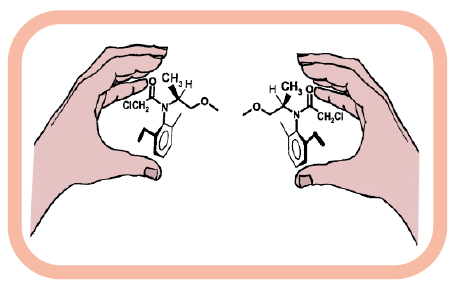
Molecular handedness of the chiral herbicide
metolachlor.
The agrochemical industry also benefits from asymmetric synthesis. Many herbicides, pesticides, and fungicides are chiral, and their activity can be enantioselective. Molecular handedness of chiral herbicide, metolachlor, is depicted in the image. Producing these compounds in their active form enhances their efficacy and reduces environmental impact.
Fine Chemicals and Materials
Chiral compounds are used in the synthesis of fine chemicals and materials with specific optical and electronic properties. These materials are essential in various applications, including liquid crystals for displays and asymmetric synthesis of fragrances and flavors.
Conclusion
Asymmetric synthesis is a vital field in modern chemistry, enabling the production of enantiomerically pure compounds essential for pharmaceuticals, agrochemicals, and advanced materials. The development of chiral catalysts and the understanding of reaction mechanisms have advanced the efficiency and scope of asymmetric synthesis. As research continues, future directions may include the integration of green chemistry principles, the development of more sustainable catalytic systems, and the application of computational methods to further enhance enantioselectivity. Understanding the fundamentals of chiral asymmetric synthesis is crucial for appreciating its impact and potential in various scientific and industrial applications. As the field progresses, it will continue to play a pivotal role in shaping the future of chemistry and related disciplines.
Further Reading
Günter Helmchen, Definition of the term asymmetric synthesis – History and revision, Chirality, 2023. https://doi.org/10.1002/chir.23536
Kagan, H. B., & Gopalaiah, K. Early history of asymmetric synthesis: who are the scientists who set up the basic principles and the first experiments? New Journal of Chemistry, 2011, 35,1933. doi:10.1039/c1nj20216b.
Pinto, M.M.M.; Fernandes, C.; Tiritan, M.E. Chiral Separations in Preparative Scale: A Medicinal Chemistry Point of View. Molecules, 2020, 25, 1931. https://doi.org/10.3390/molecules25081931.
https://en.wikipedia.org/wiki/Chirality_timeline
Reyes, E.; Prieto, L.; Milelli, A. Asymmetric Organocatalysis: A Survival Guide to Medicinal Chemists. Molecules 2023, 28, 271. https://doi.org/10.3390/molecules28010271
https://www.nobelprize.org/prizes/chemistry/2001/summary
https://www.nobelprize.org/prizes/chemistry/2021/popular-information.
Hans-Ulrich Blaser, Enantioselective Catalysis in Fine Chemicals Production, Chemical Communications, 2003, 34, 293-6. DOI:10.1039/B209968N.
Svetlana B. Tsogoeva, A Noble Quest for Simplicity in the Chiral World, N Engl J Med., 2021, 385:2579-2581 DOI: 10.1056/NEJMcibr2116228.
List, B.; Yang, J.W. The Organic Approach to Asymmetric Catalysis. Science, 2006, 313, 1584–1586. https://sci-hub.se/10.1126/science.1131945.
MacMillan, D.W.C. The advent and development of organocatalysis. Nature, 2008, 455, 304–308.
Reyes, E.; Prieto, L.; Milelli, A. Asymmetric Organocatalysis: A Survival Guide to Medicinal Chemists. Molecules, 2023, 28, 271.
https://doi.org/10.3390/molecules28010271.
Chirotechnology: Industrial synthesis of optically active compounds,” by: Roger A. Sheldon, New York: Marcel Dekker, Inc., 1993, ISBN: 0-8247-9143-6.
Biswa Mohan Sahoo and Bimal Krishna Banik, Organocatalysis: Trends of Drug Synthesis in Medicinal Chemistry, Current Organocatalysis, 2019, 6, 92 – 105. doi: 10.2174/2213337206666190405144423.
Pranshu Vishwakarma and Abhishek Verma, A review on asymmetric organocatalysis historical works and present scenario, International Journal of Creative Research Thoughts, 2024, 12(5):2320-2882, doi:10.1729/Journal.39332.
https://www.ncbi.nlm.nih.gov/pmc/articles/PMC9805200/pdf/CHIR-34-1403.pdf
Well illustrated article in this space. Nobel prize 2021 section is informative too.
Thank you for your kind words! I’m glad you found the article and the Nobel Prize 2021 section informative. It’s always rewarding to know that the content resonates with readers like you.