The intersection of chirality and medicine is a compelling narrative that reveals both the promise and peril of molecular asymmetry. From its earliest applications in pharmacology to the tragic consequences of overlooking enantiomeric differences, the role of chirality in medicine underscores the profound impact of stereochemistry on human health. This chapter delves into the breakthroughs, challenges, and lessons learned as chirality became a cornerstone of drug development.
Early Discoveries: The Physiological Impact of Chirality
The connection between chirality and biological activity was first noted in the late 19th century. In 1903, Arthur Robertson Cushny published groundbreaking research showing that the enantiomers of certain compounds, such as atropine and hyoscyamine, elicited different physiological effects. Cushny’s work provided the first clear examples of enantiospecificity -the phenomenon where one enantiomer interacts favorably with biological targets while its mirror image does not.
Cushny’s findings were further supported by Paul Ehrlich’s studies on the optical isomers of cocaine in 1894. Ehrlich observed that (+)-cocaine acted more rapidly and with greater intensity than its (-)-enantiomer. This discovery highlighted the importance of stereochemistry in drug action and marked the beginning of chirality’s integration into pharmacology and emergence of the new field Chiral Pharmacology.
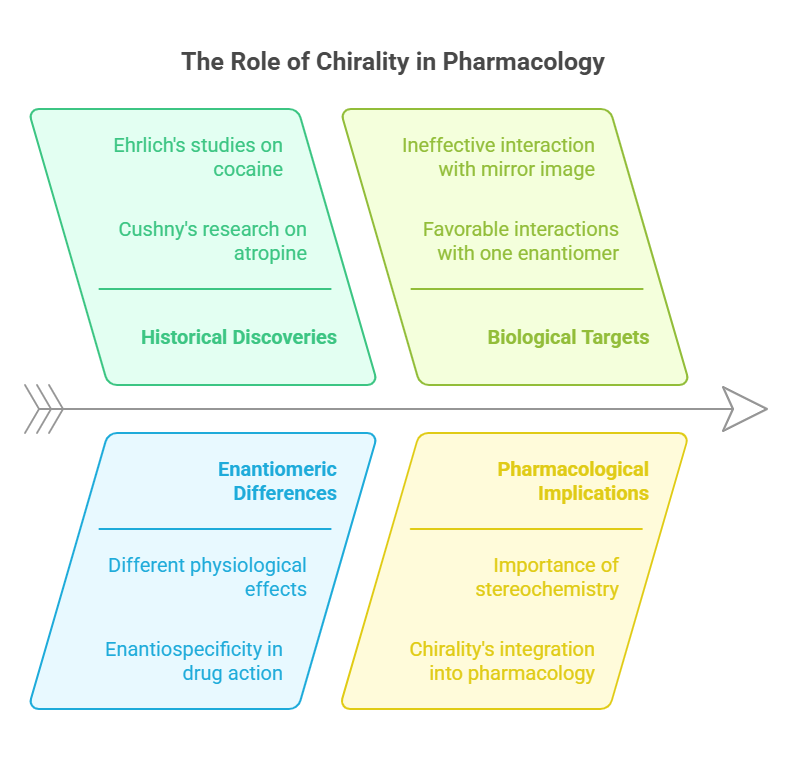
The Tragedy of Thalidomide: A Turning Point
Despite early awareness of enantiospecificity, the pharmaceutical industry largely overlooked the significance of chirality until the mid-20th century. This oversight culminated in one of medicine’s greatest tragedies: the thalidomide disaster.
Introduced in the late 1950s as a sedative and treatment for morning sickness, thalidomide was marketed as a safe and effective drug. However, it was later discovered that the drug’s two enantiomers had drastically different effects. While the (R)-thalidomide enantiomer provided therapeutic benefits, the (S)-enantiomer caused severe birth defects, including phocomelia (limb malformations). Since thalidomide was administered as a racemic mixture containing both enantiomers, its devastating consequences affected thousands of newborns worldwide. <https://chiralpedia.com/blog/thalidomide-tragedy-the-story-and-lessons/>; <https://chiralpedia.com/blog/thalidomide/>
The thalidomide tragedy prompted a paradigm shift in pharmaceutical research and regulation. Scientists and regulatory bodies recognized the necessity of understanding and controlling chirality in drug development. This realization led regulatory agencies to the establishment of stringent guidelines for evaluating the enantiomeric composition of pharmaceuticals, ultimately improving drug safety.
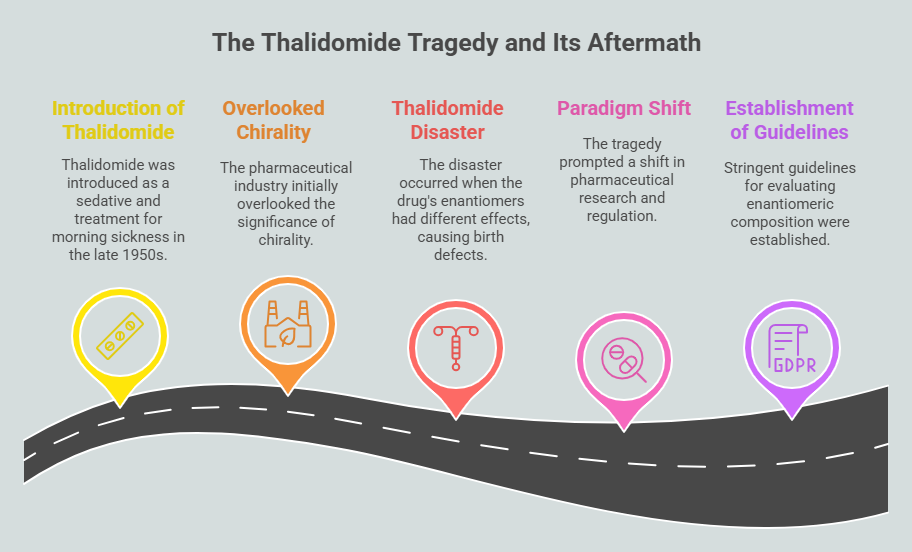
The Rise of Enantioselective Drug Development
In the aftermath of the thalidomide disaster, the pharmaceutical industry embraced enantioselective synthesis and analysis. Advances in synthetic chemistry, particularly the development of chiral catalysts and reagents, enabled the production of enantiomerically pure drugs. Techniques such as asymmetric hydrogenation, pioneered by William Knowles & Ryōji Noyori, and asymmetric oxidation innovated by K. Barry Sharpless revolutionized the field, earning all the three scientists the Nobel Prize in Chemistry in 2001.
One of the earliest successes in enantioselective drug development was the production of (+)-epinephrine, the active enantiomer of adrenaline. This breakthrough demonstrated the therapeutic advantages of isolating and administering the biologically active enantiomer. Subsequent examples, such as the selective beta-blocker (+)-propranolol and the anti-inflammatory drug (S)-ibuprofen, further underscored the importance of chirality in optimizing drug efficacy and minimizing side effects.
Case Studies in Chiral Drug Development
Propranolol: Developed in the 1960s, propranolol was the first beta-blocker to gain widespread clinical use. Although it was initially marketed as a racemic mixture, researchers soon discovered that its (S)-enantiomer (chiral switch) exhibited superior beta-adrenergic blocking activity. This finding spurred efforts to develop enantiomerically pure beta-blockers, leading to safer and more effective cardiovascular therapies.
Albuterol: Albuterol, a bronchodilator used to treat asthma, provides another compelling example of enantiospecificity. The (R)-enantiomer, known as levalbuterol, is responsible for the drug’s therapeutic effects, while the (S)-enantiomer contributes to side effects. By isolating and administering (R)-albuterol, pharmaceutical companies were able to improve the drug’s safety profile.
Omeprazole: The proton pump inhibitor omeprazole, used to treat acid reflux and peptic ulcers, is a racemic mixture. However, its (S)-enantiomer, marketed as esomeprazole, exhibits enhanced bioavailability and therapeutic efficacy. This advancement highlights the role of chirality in refining drug formulations.
Challenges in Chiral Drug Development
While the benefits of enantiomerically pure drugs are clear, their development poses significant challenges. The synthesis of single-enantiomer compounds often requires complex and costly techniques. Additionally, analyzing and characterizing chiral molecules demands advanced instrumentation, such as chiral chromatography and nuclear magnetic resonance (NMR) spectroscopy.
Regulatory requirements add another layer of complexity. Agencies such as the U.S. Food and Drug Administration (FDA) and the European Medicines Agency (EMA) mandate rigorous evaluation of enantiomeric purity, pharmacokinetics, and toxicology. These requirements ensure drug safety but also increase development timelines and costs.
Chirality in Natural Products and Biotechnology
Many natural products – compounds derived from plants, fungi, and microorganisms – are chiral. Their stereochemistry often determines their biological activity, making them valuable sources of pharmaceuticals. For example:
- Taxol (Paclitaxel): A chiral compound derived from the Pacific yew tree, taxol is a potent anticancer agent that stabilizes microtubules and inhibits cell division.
- Erythromycin: This macrolide antibiotic’s stereochemistry is crucial for binding bacterial ribosomes and inhibiting protein synthesis.
- Vancomycin: A glycopeptide antibiotic, vancomycin’s chiral structure enables it to target bacterial cell wall synthesis selectively.
Advances in biotechnology have further expanded the potential of chiral drugs. Techniques such as enzyme-mediated synthesis and recombinant DNA technology allow for the production of complex chiral molecules with high specificity and efficiency. These innovations have accelerated the discovery and development of new therapeutics.
Chirality Beyond Pharmaceuticals: Diagnostic and Imaging Agents
The applications of chirality extend beyond therapeutics. Chiral molecules play a vital role in diagnostic and imaging technologies, where their stereochemistry influences their interaction with biological systems. Examples include:
- Fluorodeoxyglucose (FDG): A radiolabeled glucose analogue used in positron emission tomography (PET) imaging. Its chirality ensures selective uptake by metabolically active tissues.
- Gadolinium-based contrast agents: Used in magnetic resonance imaging (MRI), these agents rely on chiral chelators to enhance stability and specificity.
Lessons Learned and Future Directions
The integration of chirality into medicine has been a journey of discovery and learning. The thalidomide tragedy served as a stark reminder of the need to understand and control stereochemistry in drug development. Subsequent advances in enantioselective synthesis and analysis have transformed pharmacology, enabling the design of safer and more effective therapeutics.
Looking ahead, several trends are shaping the future of chiral medicine:
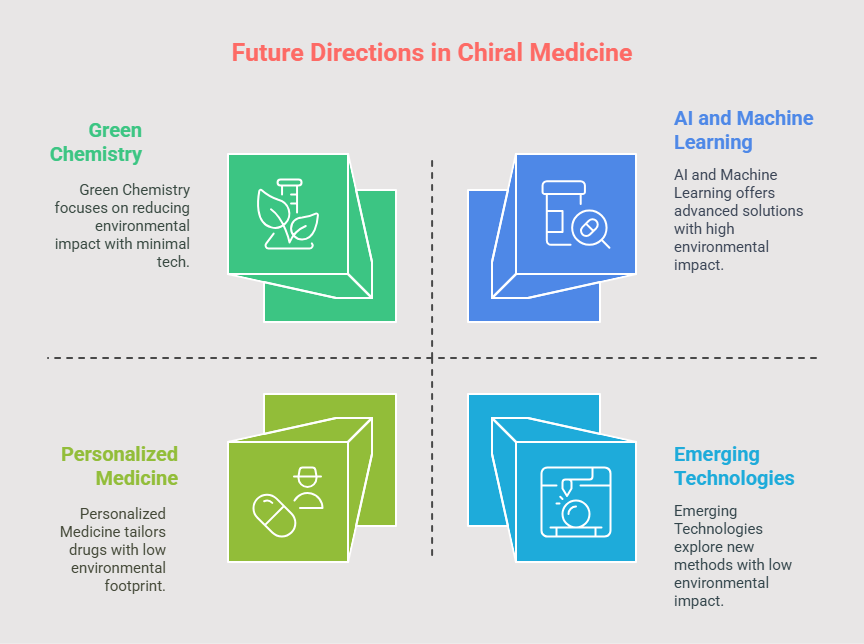
Conclusion: A Tale of Caution and Progress
The history of chirality in medicine is a testament to the interplay between scientific discovery and human health. From Cushny’s early observations to the thalidomide tragedy and the rise of enantioselective synthesis, each milestone has shaped the field of pharmacology. Today, chirality is recognized as a fundamental consideration in drug development, guiding the creation of safer, more effective therapies.
As we continue to explore the complexities of molecular asymmetry, the lessons of the past remind us of the importance of diligence, innovation, and responsibility. The story of chirality in medicine is far from over, with new challenges and opportunities on the horizon. By embracing the principles of stereochemistry, we can continue to advance the frontiers of healthcare, improving lives and transforming medicine.
References
Cushny AR (1903). “Atropine and the Hyoscyamines: A Study of the Action of Optical Isomers.” The Journal of Physiology.
Ehrlich P, Einhorn A (1894). “On the Physiological Effects of Cocaine Optical Isomers.” Berichte der Deutschen Chemischen Gesellschaft.
Sheldon RA (1993). Chirotechnology: Industrial Synthesis of Optically Active Compounds. Marcel Dekker.
Noyori R (2002). “Asymmetric Catalysis in Organic Synthesis.” Angewandte Chemie International Edition.
FDA (1992). “Policy Statement for the Development of New Stereoisomeric Drugs.” U.S. Food and Drug Administration.
Knowles WS, Noyori R (2001). “Nobel Lectures: Asymmetric Hydrogenation.” Nobelprize.org.
Smith SW (2009). “Chiral Toxicology: It’s the Same Thing… Only Different.” Toxicological Sciences.
Chirality in Drug Research, Eric Francotte (Editor), Wolfgang Lindner (Editor), Raimund Mannhold (Series Editor), Hugo Kubinyi (Series Editor), Gerd Folkers (Series Editor). ISBN: 978-3-527-31076-0. 2006. https://www.wiley.com/en-us/Chirality+in+Drug+Research-p-9783527310760