Lead
Chirality – a concept rooted in the mirror-image asymmetry observed in nature – stands as a cornerstone of chemistry and pharmaceutical sciences. This journey begins in the early 19th century, where the interplay of physics, chemistry, and biology unveiled the mysterious world of chiral molecules. These pioneering explorations profoundly changed our understanding of molecular structures and their pivotal roles in biological and pharmaceutical applications.
Étienne-Louis Malus: The First Step into the Polarized World
In 1809, French physicist Étienne-Louis Malus observed a peculiar phenomenon while studying light reflected off glass surfaces. He discovered that the reflected light became “polarized,” exhibiting unique directional properties. This revelation laid the groundwork for understanding the orientation of light waves and their interactions with matter.
Malus faced significant hurdles – chief among them, skepticism from contemporaries who struggled to grasp the practical implications of polarization. Furthermore, the lack of advanced optical tools limited the precision of his observations. Malus persisted through these challenges, setting the stage for subsequent explorations of light and symmetry. His findings would later influence scientists attempting to uncover the nature of molecular interactions and asymmetry.
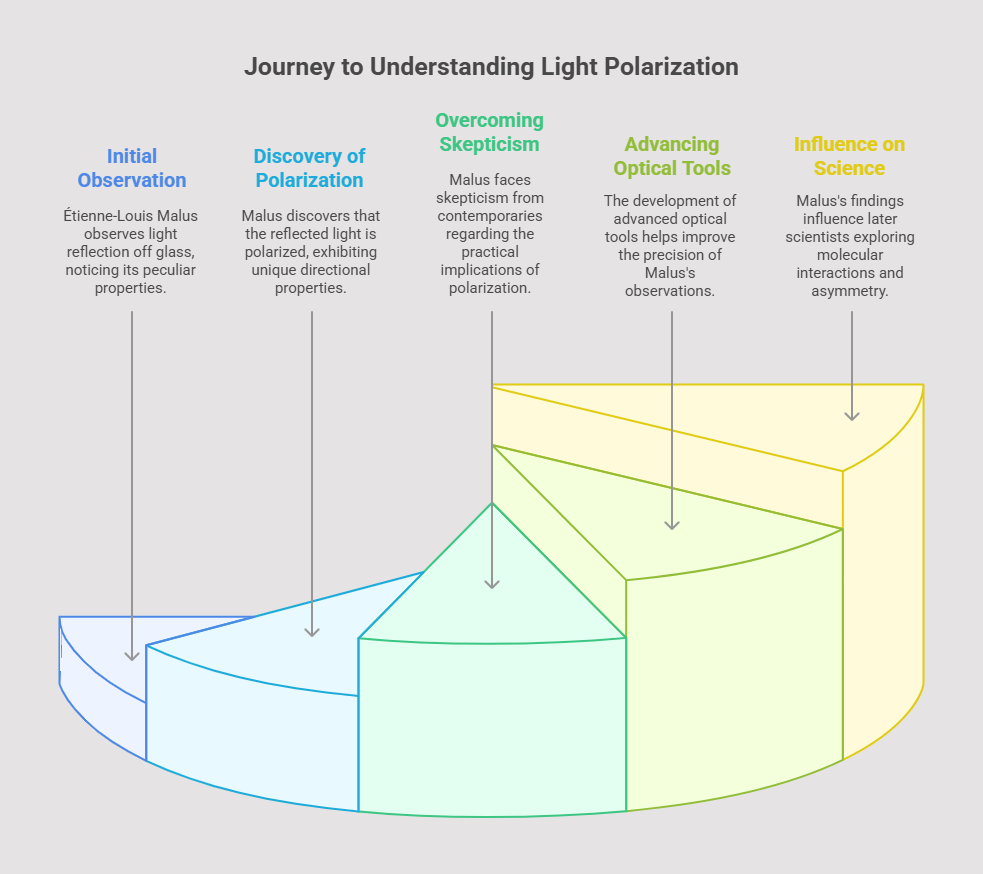
Jean-Baptiste Biot: Connecting Light and Molecules
Building on Malus’ discovery, Jean-Baptiste Biot expanded the investigation into polarized light. In 1812, he demonstrated that certain substances, such as quartz and sugar solutions, could rotate the plane of polarized light. This phenomenon, termed “optical rotation,” varied with the thickness of the material and the concentration of the solution.
Biot’s experiments were arduous. The instruments of his time lacked the accuracy needed for such delicate measurements, leading to frustrations over reproducibility. His meticulous approach, however, yielded groundbreaking results. By correlating molecular structure with optical activity, Biot provided the first empirical link between molecular asymmetry and physical phenomena. This work not only solidified the foundation of stereochemistry but also sparked widespread interest in the molecular basis of optical activity.
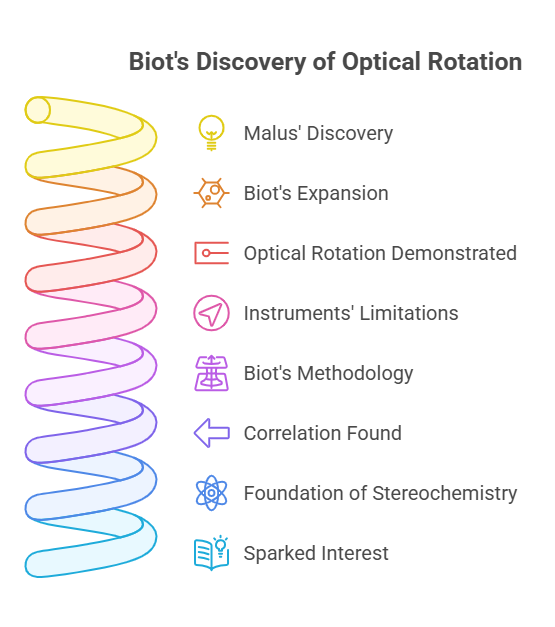
Louis Pasteur: Crystals that Changed Chemistry Forever
In 1848, Louis Pasteur, then a young chemist, took the next monumental step in understanding chirality. While studying tartaric acid crystals derived from wine-making residues, Pasteur noticed two distinct types of crystals under his microscope. These were mirror images of each other. With extraordinary precision, he manually separated the crystals using tweezers, marking the first experimental isolation of enantiomers—molecules that are non-superimposable mirror images of one another.
Pasteur’s discovery was revolutionary but met with fierce resistance. Critics dismissed his findings as anomalies, while the painstaking nature of his work made replication difficult. Despite these challenges, Pasteur’s insights into molecular asymmetry would prove transformative, particularly in understanding the biochemical specificity of life. He hypothesized that this asymmetry played a role in biological activity, an idea later validated in pharmacology, where chirality often dictates a drug’s efficacy and safety.
Pasteur’s work also hinted at broader implications: why life itself exhibits such profound chirality. His hypothesis that biological systems preferentially utilize one enantiomer over another became a fundamental concept in biochemistry and molecular biology. This idea extended beyond theory, finding practical applications in modern pharmaceutical development.
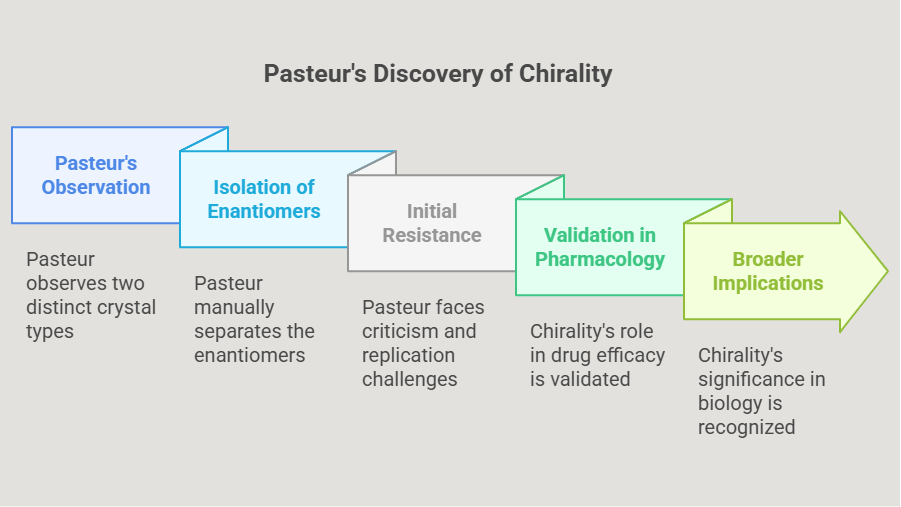
Challenges of an Asymmetric Revolution
The early 19th century was an era of scientific conservatism. Concepts like molecular asymmetry faced resistance because they contradicted established notions of molecular uniformity. Moreover, the rudimentary tools available to Malus, Biot, and Pasteur forced them to rely on labor-intensive methods and innovative thinking. Their perseverance in the face of these limitations underscores the magnitude of their achievements.
For Malus, the challenge lay in convincing a skeptical scientific community that polarized light was more than an optical curiosity. Biot struggled with the reproducibility of his data, often frustrated by the inadequacy of his tools. Pasteur’s work, though celebrated today, was met with doubt and intense scrutiny during his lifetime, forcing him to repeatedly defend and refine his methods.
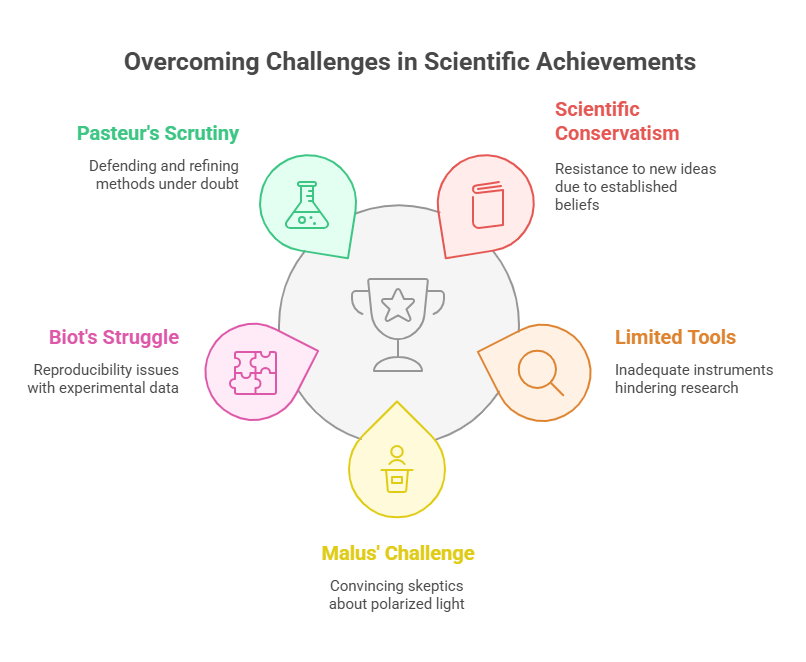
The Impact of Early Breakthroughs
The contributions of Malus, Biot, and Pasteur transcended their time, laying the foundation for stereochemistry and its critical applications in modern science. Malus’ work on polarized light inspired the development of advanced optical instruments, which became essential in analyzing molecular structures. Biot’s studies on optical rotation found early applications in industries like sugar refinement and later became indispensable in pharmaceutical research. Pasteur’s discovery of enantiomers provided the conceptual framework for understanding chirality in biological systems, influencing the design of drugs, enzymes, and materials.
These breakthroughs also catalyzed further advancements. By the late 19th century, chemists such as Jacobus Henricus van’t Hoff and Joseph Achille Le Bel built upon Pasteur’s work to develop stereochemical models of molecular structure. The field of stereochemistry – rooted in the pioneering work of Malus, Biot, and Pasteur – emerged as a vital area of research, bridging chemistry and biology.
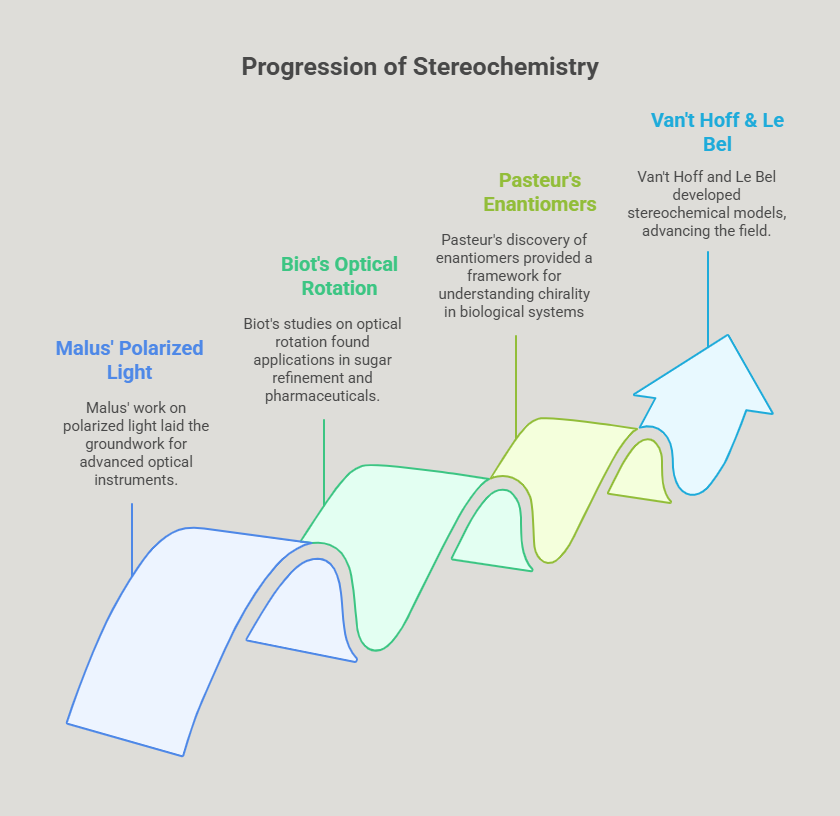
Chirality: Nature’s Blueprint for Life
Chirality is a defining characteristic of many biological molecules, including DNA, proteins, and carbohydrates. This asymmetry is not arbitrary but fundamental to molecular interactions. For example, the chiral molecule limonene exists in two forms: one smells like oranges, while the other smells like lemons. Similarly, in pharmacology, the efficacy and safety of drugs often depend on their chiral form. The infamous thalidomide tragedy of the 1960s underscored the life-and-death importance of understanding chirality, as one enantiomer caused severe birth defects while the other provided therapeutic benefits.
The pharmaceutical industry, in particular, owes much to the early pioneers of chirality. Understanding enantiomeric purity—the prevalence of one enantiomer over another – became crucial for drug development. Today, methods like chiral chromatography and advanced spectroscopy owe their origins to the foundational principles established by Malus, Biot, and Pasteur.
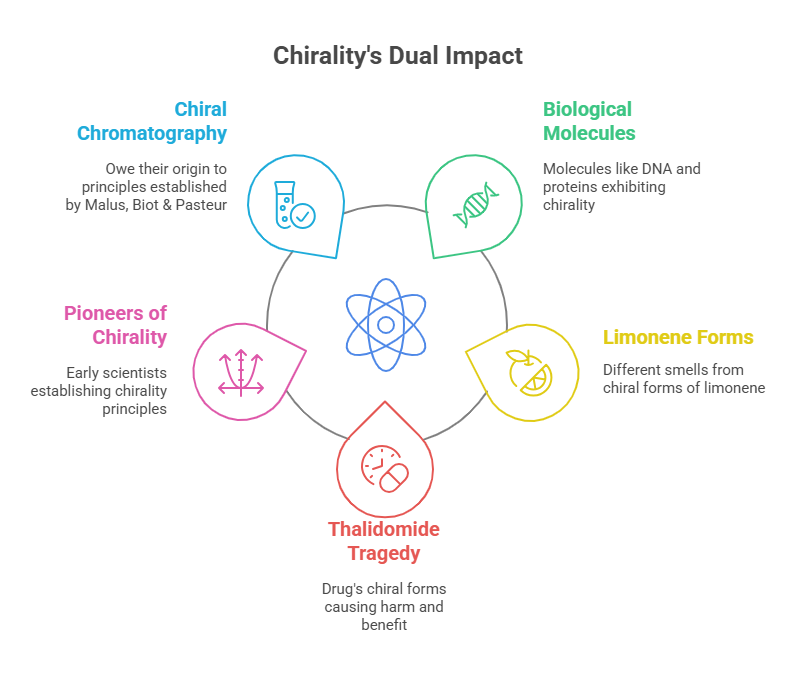
Conclusion: A Legacy of Discovery and Innovation
The origins of chirality – from Étienne-Louis Malus’ exploration of polarized light to Louis Pasteur’s discovery of molecular asymmetry -highlight the transformative power of curiosity, perseverance, and scientific rigor. These pioneers overcame skepticism and technical limitations to reveal the asymmetric nature of molecules, a revelation that continues to shape chemistry, biology, and pharmaceutical sciences. Their work not only provided a deeper understanding of molecular interactions but also laid the groundwork for innovations that would save lives and advance human knowledge.
As we look back on their contributions, it becomes clear that the story of chirality is not just one of scientific discovery but also of human ingenuity. The challenges they faced and the breakthroughs they achieved resonate in today’s research and applications, proving that the pursuit of knowledge knows no bounds.
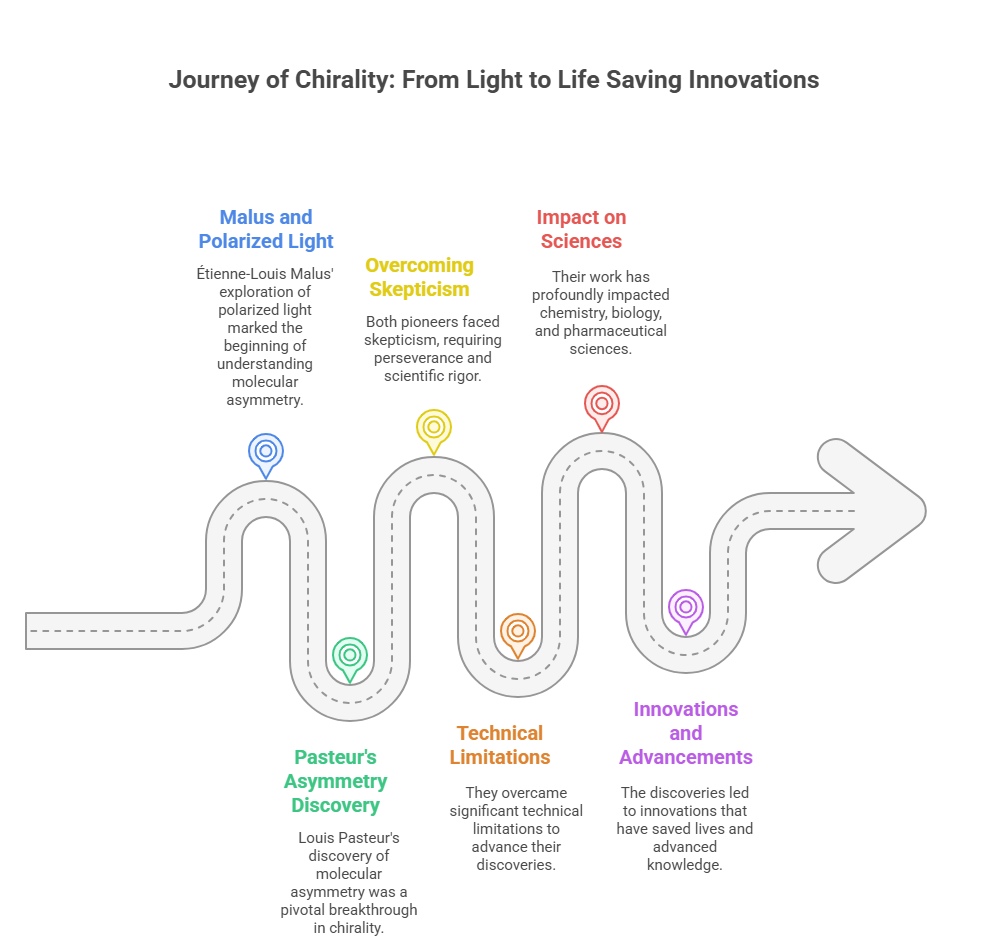
References and Further Reading
Malus EL (1809). “Sur une propriété de la lumière réfléchie.” Mémoires de physique et de chimie de la Société d’Arcueil.
Biot JB (1812). “Sur de nouveaux rapports qui existent entre la réflexion et la polarisation de la lumière des corps cristallisés.” Mémoires de la classe des sciences mathématiques et physiques.
Pasteur L (1848). “Recherches sur les propriétés optiques des corps cristallisés.” Comptes rendus de l’Académie des Sciences.
Crossley R (1992). “The relevance of chirality to the study of biological activity.” Tetrahedron. 48(38): 8155–8178. https://doi.org/10.1016/S0040-4020(01)80486-5
Sheldon RA (1993). Chirotechnology: Industrial Synthesis of Optically Active Compounds. Marcel Dekker. ISBN 9780824791438 / 0824791436
https://en.wikipedia.org/wiki/Chirality_timeline