Introduction
Molecules can come in two shapes that mirror each other, in the same way as our left and right hands. This attribute, called chirality, can be found in biological molecules like sugars and proteins This property, often referred to as molecular handedness, plays a crucial role in chemistry, biology, and pharmacology. In this article, we will explore the basics of chirality, examine examples of chiral and achiral molecules, and delve into the methods used to determine the chirality of molecules.
Explanation of Chiral Molecules
Chirality in molecules is a geometric property where a molecule cannot be superimposed on its mirror image. These non-superimposable mirror images are called enantiomers. The concept of chirality is rooted in the presence, of a chiral center (also referred to as a stereo-center or stereogenic center), usually a carbon atom that is bonded to four different substituents. This asymmetry prevents the molecule from being identical to its mirror image, thus giving rise to chirality.
In geometry, a figure is chiral (and said to have chirality) if it is not identical to its mirror image, or, more precisely, if it cannot be mapped to its mirror image by rotations and translations alone. An object that is not chiral is said to be achiral.
Chirality is distinct from achirality, where molecules are superimposable on their mirror images. Achiral molecules often have a plane of symmetry, which divides the molecule into two identical halves. In contrast, chiral molecules lack such symmetry, resulting in distinct left- and right-handed forms.
To illustrate, consider a carbon atom bonded to four different groups: hydrogen (H), methyl (CH3), hydroxyl (OH), and ethyl (C2H5). This carbon atom becomes a chiral center, creating two enantiomers that are mirror images of each other but cannot be superimposed. This property is analogous to our hands; although they are mirror images, the left hand cannot be perfectly aligned with the right hand.
Examples of Chiral and Achiral Molecules
The below image depict a pair of hands as an example of chiral objects and a sphere, such as a ball, as an achiral object, it being identical to its mirror image. [Source: https://www.makingmolecules.com/blog/chirality]
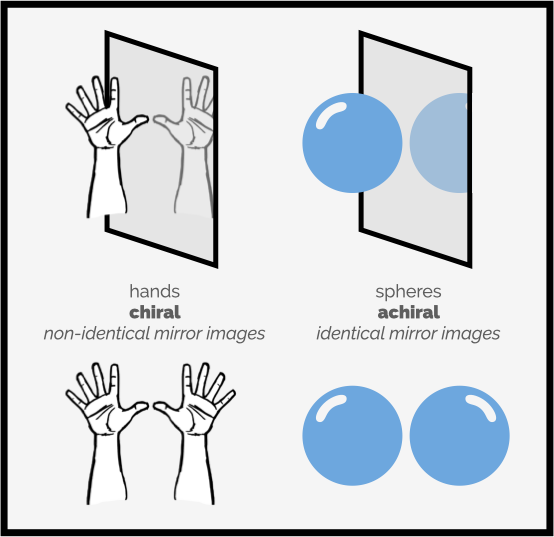
This image contrasts chiral and achiral objects through the example of hands and spheres. Hands are chiral because their mirror images are non-identical, illustrating non-superimposable mirror images. In contrast, spheres are achiral as their mirror images are identical and superimposable. This distinction is fundamental in understanding symmetry and molecular structures in chemistry and biology.
Methane (CH4) and ethane (C2H6) are examples of achiral molecules. Methane, with four identical hydrogen atoms bonded to a central carbon, is perfectly symmetrical and superimposable on its mirror image. Similarly, ethane consists of two carbon atoms bonded to six hydrogen atoms in a symmetrical arrangement, making it achiral.
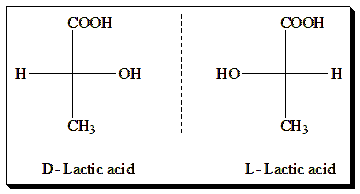
This image showcases the chirality of lactic acid molecules, presenting D-lactic acid and L-lactic acid. These enantiomers are non-superimposable mirror images of each other, highlighting the concept of chirality in organic chemistry. Chirality is critical in biochemistry, as the two forms can have different biological activities and properties.
Chirality is prevalent in many naturally occurring and synthetic molecules. A quintessential example of a chiral molecule is lactic acid, which has a chiral center at the carbon atom bonded to hydrogen, hydroxyl, and two other carbon-containing groups. Lactic acid exists in two enantiomeric forms: L-lactic acid and D-lactic acid, which are mirror images but not superimposable. Mirror-image twins of lactic acid are depicted in Fischer projection formula in the Figure (right-hand side)
Another prominent example is glucose, a simple sugar that plays a vital role in energy metabolism. Glucose contains five chiral centers leading to the existence of several stereoisomers , each with distinct biological properties. The D-isomer of glucose is the most common form found in nature and is crucial for cellular respiration.
Molecular Shape matters !!!
Essentially mirror-image twins (enantiomers) differ in their configuration. In other words, simply they differ in their shape. Definition of shape in molecular pharmacology does encompass structural features like depth, size and surface. Molecular shape is of great importance and has profound influence on biological processes. Hence chirality that dictates molecular shape is particularly significant in the realm of pharmaceuticals.

(This image illustrates the concept of molecular recognition and chirality using both a simple toy analogy and complex protein structures. [Source: doi: 10.1016/j.tips.2008.12.001].
A. A toy with various shaped holes and corresponding shapes illustrates the concept of molecular fit and recognition, similar to how molecules interact based on shape and orientation.
B. A protein complex structure demonstrates the specific interactions between different protein subunits, highlighting how chirality and shape complementarity are crucial for biological function.
C. A detailed molecular model shows how different molecules fit into a larger macromolecule, emphasizing the
importance of chirality in determining the precise fit and interaction within biological systems, where size and shape complementarity may be essential in addition to favorable electrostatic and steric interactions, to fulfill the “lock and key” or “induced fit” hypotheses.
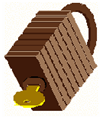
To give an idea of the impact of chirality in pharmacological consequences two case studies are presented. More critical analysis of this aspect will be dealt in the forthcoming blog on “Chirality in Pharmaceuticals: The Role of Handedness in Medicine“.
Case Study 1: Thalidomide
The thalidomide disaster in the 1960s underscored the importance of chirality in drug design. Thalidomide, a drug prescribed to pregnant women to alleviate morning sickness, existed as two enantiomers. One enantiomer had the desired sedative effect, while the other caused severe birth defects. This tragedy highlighted the need for enantiomerically pure drugs, where only the therapeutically beneficial enantiomer is administered.
Case study 2: Ibuprofen
Ibuprofen, a common nonsteroidal anti-inflammatory drug (NSAID), is another example where chirality plays a role. Ibuprofen is sold as a racemic mixture, containing both enantiomers. However, only one enantiomer is responsible for the drug’s anti-inflammatory effects. Pure enantiomer of ibuprofen called “Dexibuprofen” is also available in the market. Understanding the chirality of drugs is crucial for their safe and effective use.
How to Determine the Chirality of a Molecule
Identifying chiral centers in molecules is the first step in determining their chirality. A chiral center, typically a carbon atom bonded to four different groups, is the hallmark of a chiral molecule. To determine chirality, chemists use various techniques that allow them to visualize and analyze the three-dimensional arrangement of atoms in a molecule.
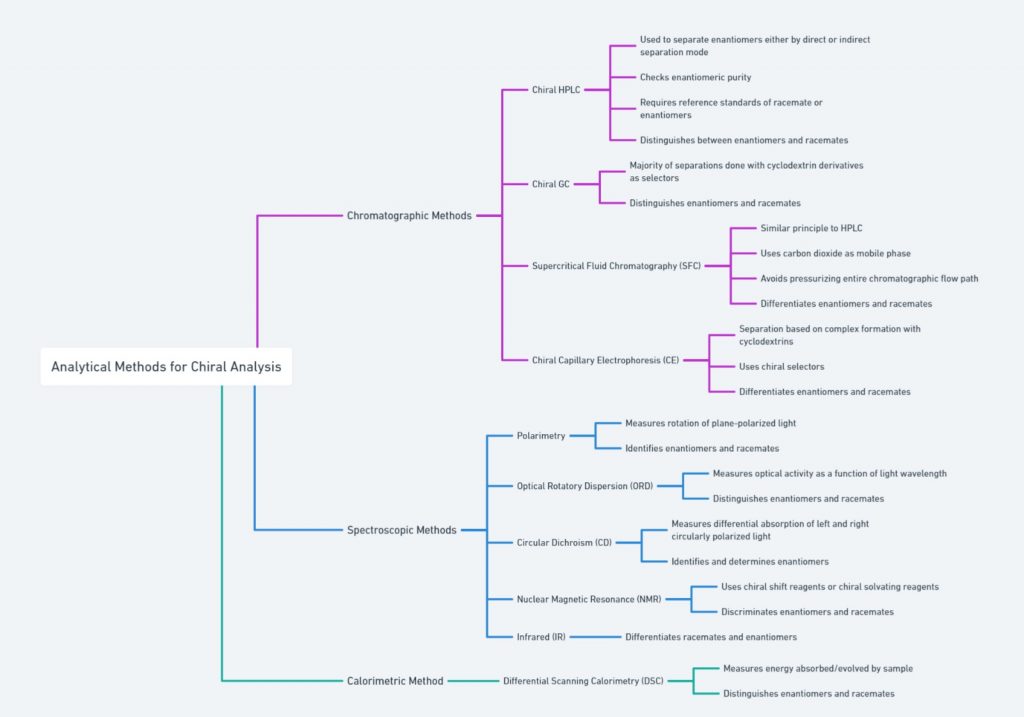
[ categorized into chromatographic, spectroscopic, and calorimetric methods]
Molecular Models
Molecular models are valuable tools for visualizing chirality. By constructing a physical (e.g. ball and stick model) or digital model of a molecule, chemists can observe the spatial arrangement of atoms and identify chiral centers. These models help in understanding the non-superimposability of enantiomers and provide a clear representation of the molecule’s three-dimensional structure.
Polarimetry/Optical rotation
Polarimetry is a technique used to measure the optical rotation of chiral substances. When plane-polarized light passes through a solution of a chiral compound, the plane of polarization is rotated. The direction and degree of this rotation, known as optical rotation, are characteristic of the specific enantiomer present. Polarimetry is commonly used to determine the enantiomeric purity of a sample and to distinguish between enantiomers.
X-ray Crystallography
X-ray crystallography provides detailed images of the molecular structure of a compound. By analyzing the diffraction pattern of X-rays passing through a crystal, chemists can determine the exact spatial arrangement of atoms within the molecule. This technique is particularly useful for identifying chiral centers and understanding the three-dimensional geometry of complex molecules.
NMR Spectroscopy
Nuclear Magnetic Resonance (NMR) spectroscopy is another powerful technique for studying chiral molecules. Chiral shift reagents can be added to the sample, inducing chemical shifts that differ between enantiomers. These shifts allow for the identification and quantification of enantiomers in a mixture. NMR spectroscopy provides detailed information about the molecular environment and is widely used in the analysis of chiral compounds.
Chiral Chromatography
Chiral chromatography, on the other hand, uses chiral stationary phases to separate enantiomers based on their interactions with the chiral environment of the column. This technique is highly effective in resolving racemic mixtures and is commonly used in the pharmaceutical industry to ensure the enantiomeric purity of drugs.
Applications and Implications of Chirality
Chirality has far-reaching implications in various scientific fields and practical applications. In biological systems, chirality plays a crucial role in molecular recognition processes. Enzymes, which are chiral themselves, often exhibit high specificity for one enantiomer of a substrate. This specificity is essential for the proper functioning of biochemical pathways.
In drug design and development, chirality is of paramount importance. Many drugs are chiral, and the therapeutic activity is often limited to one enantiomer. The development of enantiomerically pure drugs ensures efficacy and reduces the risk of side effects associated with the inactive or harmful enantiomer. Regulatory agencies now require rigorous testing and approval processes for each enantiomer of a chiral drug.
Chirality also finds applications in industrial processes. Chiral catalysts are used to produce enantiomerically pure compounds, essential for the synthesis of pharmaceuticals, agrochemicals, and other fine chemicals. The development of efficient chiral catalysts has revolutionized synthetic chemistry, enabling the production of complex chiral molecules with high selectivity and yield. For more insight to this topic refer chiralpedia.com @ the following link <https://chiralpedia.com/chiral-synthesis.php>.
Furthermore, chirality has potential applications in the emerging fields of materials science and nanotechnology. Chiral materials exhibit unique optical, electronic, and mechanical properties, making them suitable for various advanced technologies. Chiral nanoparticles, for instance, can be used for targeted drug delivery, imaging, and the development of novel materials with tailored properties.
Conclusion
Chirality, the property of molecular handedness, is a fundamental concept that shapes the structure and function of molecules. Understanding chirality is essential for deciphering the complexities of chemical and biological systems. From its basic principles to its applications in drug design and industrial processes, chirality continues to play a pivotal role in scientific research and technological advancements.
As our understanding of chirality deepens, new opportunities for innovation and discovery arise. The study of chiral molecules will undoubtedly remain a cornerstone of chemistry and biology, driving progress in fields ranging from medicine to materials science. By appreciating the significance of chirality, we can unlock the potential of molecular handedness to shape the future of science and technology.
Further Reading
Kortagere S, Krasowski MD, Ekins S. The importance of discerning shape in molecular pharmacology. Trends Pharmacol Sci. 2009 Mar; 30(3): 138–147.. doi: 10.1016/j.tips.2008.12.001. Epub 2009 Jan 31. PMID: 19187977; PMCID: PMC2854656.
https://chiralpedia.com/blog/dexibuprofen
Francotte, Eric; Lindner, Wolfgang (2006). Chirality in drug research. Eric Francotte, W. Lindner. Weinheim: Wiley-VCH. p. 205. ISBN 978-3-527-60943-7. OCLC 163578005.
Cahn, B. S.; lngold, C. K; Prelog, V (1956). “The specification of asymmetric configuration in organic chemistry”. Experientia. 12 (3): 81–88. doi:10.1007/BF02157171. S2CID 43026989
Mislow, Kurt; Siegel, Jay (1984). “Stereoisomerism and local chirality”. Journal of the American Chemical Society. 106 (11): 3319–3328. doi:10.1021/ja00323a043
https://chiralpedia.com/blog/d-l-system-naming-the-left-or-right-hand-side/
Lien Ai Nguyen, Hua He, and Chuong Pham-Huy, Chiral drugs, https://www.ncbi.nlm.nih.gov/pmc/articles/PMC3614593/
Chiral drugs. Wikipedia, Wikipedia Foundation, 21/05/2022. https://en.wikipedia.org/wiki/Chiral_drugs
Francotte, Eric; Lindner, Wolfgang (2006). Chirality in drug research. Eric Francotte, W. Lindner. Weinheim: Wiley-VCH. ISBN 978-3-527-60943-7. OCLC 163578005.
“Chiral analysis” Wikipedia, Wikimedia Foundation, 21/05/2022. https://en.wikipedia.org/wiki/Chiral_analysis
K. Valliappan, Isomerism and isomeric drugs, Indian drugs, 33(3), 98-106, 1996 and references therein. https://chiralpedia.com/pdf/Indian-Drugs-Isomerism-and-Isomeric-Drugs.pdf
“Chiral switch” Wikipedia, Wikimedia Foundation, 22/05/2022. https://en.wikipedia.org/wiki/Chiral_switch
Other Blogs on #ChiralityonFriday
1. Introduction to Chirality: Understanding the Basics
2. Molecular Handedness: How Chirality Shapes Molecules
3. Chirality in Nature: From DNA to Snail Shells
4. The Chemistry of Taste and Smell: How Chirality Affects Senses
5. Chiral Chemistry in Everyday Life: Hidden Handedness Around Us
7. Chirality-in-materials-science-designing-with-handedness/
8. Chirality in Space: Cosmic Asymmetry and the Origins of Life
9. The Future of Chirality: Innovations and Emerging Trends
10. Chirality and You: Understanding and Appreciating Molecular Handedness