Introduction
Chirality, derived from the Greek word for “hand,” is a property of asymmetry that is ubiquitous in nature. Just as our hands are mirror images but not identical, many biological molecules exhibit this fascinating characteristic. Chirality is not just a molecular curiosity; it profoundly influences the structure and function of DNA, proteins, and enzymes, as well as various physiological and behavioral traits in plants and animals. In this blog, we will explore the role of chirality in biological systems, its presence in plants and animals, and the broader implications of chirality in nature.
Chirality in Biological Systems
The reason why chirality is important as biological activity is that molecular symmetry dominates biological events. Although chirality is not essential for bioactivity, there are great differences in the activities of enantiomers in bioactive molecules with stereogenic centers such as drugs, flavoring, and food additives. The molecular components of living organisms are mostly chiral and these molecules have a dominant role in their interaction with bioactive substances. Read more @ https://biyokimya.vet/en-gb/the-importance-of-chirality-in-biological-systems/
DNA: The Double Helix
DNA, the blueprint of life, is a prime example of chirality in biological systems. The double helix structure of DNA is inherently chiral, with the two strands winding around each other in a right-handed spiral. This right-handed chirality is crucial for the stability and functionality of DNA. During replication and transcription, the enzymes involved must recognize and interact with the chiral structure of DNA, ensuring the fidelity of genetic information transfer. The chiral nature of DNA is not just a structural feature but also plays a vital role in the molecular mechanisms that sustain life.
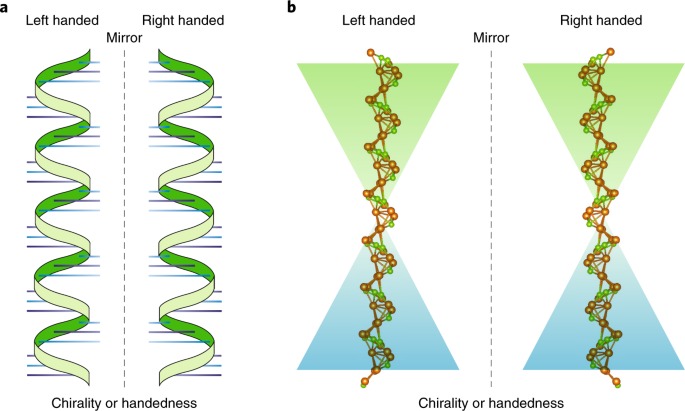
(a) Illustrations of left-handed and right-handed DNA helices. The left-handed helix (left) and right-handed helix (right) show the distinct direction in which the DNA strands twist. The mirror line in the center highlights the non-superimposable nature of the two forms, demonstrating their chirality.
(b) Molecular representations of left-handed and right-handed DNA. The left-handed form (left) and the right-handed form (right) emphasize the structural differences at the atomic level. The colored background gradients further accentuate the contrast between the two chiral forms.
The chirality, or handedness, of DNA is a fundamental characteristic that significantly impacts its biological interactions and functions. The right-handed B-DNA is the most prevalent form in nature, essential for processes such as replication and transcription, while the left-handed Z-DNA, though less common, plays roles in specific biological contexts.
Proteins: The Building Blocks of Life
Proteins, composed of chiral amino acids, are the workhorses of biological systems. The chiral nature of amino acids leads to the formation of proteins with specific three-dimensional structures, which are essential for their biological activity. The chirality of proteins influences their folding, stability, and interactions with other molecules. For instance, enzymes, which are proteins, have chiral active sites that can selectively bind to chiral substrates. This specificity is crucial for the catalytic efficiency and regulatory mechanisms of enzymes. The biological functions of proteins, ranging from structural support to catalysis and signal transduction, are intimately linked to their chiral properties.
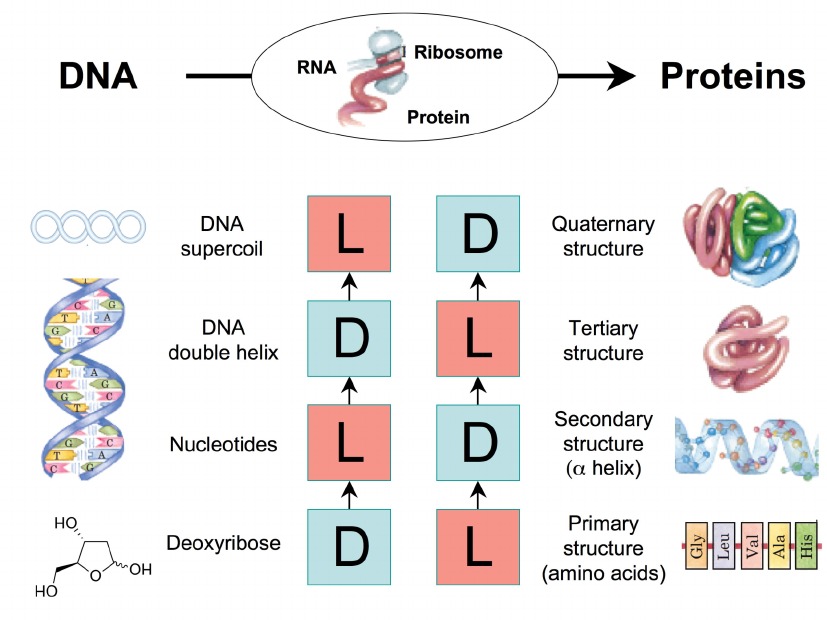
This figure illustrates the relationship between the chirality of DNA and proteins, emphasizing how chirality is preserved and propagated from genetic material to functional protein structures. DNA exists as a double helix, with chiral components at various levels, including nucleotides in the D (right-handed) configuration. The genetic information encoded in DNA is transcribed into RNA and subsequently translated into proteins by ribosomes. Proteins themselves exhibit chirality, starting with their primary structure, composed of amino acids predominantly in the L (left-handed) configuration. This chirality is maintained through the protein’s secondary structure (α-helix), tertiary structure (three-dimensional folding of a single polypeptide chain), and quaternary structure (assembly of multiple polypeptide subunits). The preservation of chirality from DNA to protein structures is crucial for the proper functioning and interactions of these biomolecules within biological systems.
Enzymes: Nature’s Catalysts
Enzymes, nature’s biological catalysts, exhibit chirality in their active sites, enabling them to discriminate between different enantiomers of a substrate. This chiral specificity is fundamental to many biochemical reactions. For example, the enzyme lactate dehydrogenase selectively catalyzes the conversion of L-lactate to pyruvate but not its D-enantiomer. Such chiral selectivity ensures the proper metabolic pathways are followed, maintaining the efficiency and regulation of cellular processes. The role of chirality in enzyme function underscores the importance of molecular handedness in biological systems.
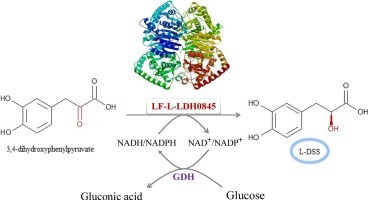
Huan Lu, Yajun Bai, Tai-ping Fan, Ye Zhao, Xiaohui Zheng, Yujie Cai,
Identification of a l-Lactate dehydrogenase with 3,4-dihydroxyphenylpyruvic reduction activity for l-Danshensu production, Process Biochemistry, Volume 72, 2018, Pages 119-123, ISSN 1359-5113, https://doi.org/10.1016/j.procbio.2018.06.011.
This figure illustrates the enzymatic conversion of 3,4-dihydroxyphenylpyruvate to L-DSS (L-Dihydroxyphenylserine) through the action of the enzyme LF-L-LDH0845, emphasizing the role of chirality in biochemical processes. The substrate, 3,4-dihydroxyphenylpyruvate, features specific chiral centers, indicated by the positions of the hydroxyl (OH) groups. The enzyme LF-L-LDH0845 catalyzes the reaction, ensuring the production of L-DSS with a particular chiral configuration. The reaction involves redox cofactors NADH/NADPH and NAD+/NADP+, which facilitate electron transfer. This process underscores the significance of chirality in enzymatic reactions, where the three-dimensional arrangement of atoms in both the substrate and product is critical for enzyme activity and the resulting biochemical pathways.
Chirality in Plants and Animals
Chirality in Plants

Plants produce a variety of chiral molecules, including alkaloids, terpenes, and flavonoids, which play crucial roles in their defense mechanisms and signaling processes. For instance, the chiral alkaloid quinine, derived from the bark of the cinchona tree, has potent antimalarial properties. Terpenes, another class of chiral molecules, contribute to the aroma and flavor of many plants and also serve as defense compounds against herbivores and pathogens. The chiral nature of these molecules affects their biological activity and interactions with other organisms, highlighting the ecological significance of chirality in the plant kingdom.
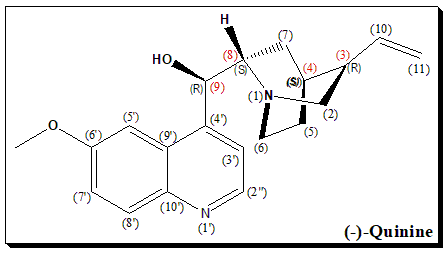
(-)-Quinine is a naturally occurring alkaloid with multiple chiral centers, denoted by the carbon atoms marked with (R) and (S) configurations. These chiral centers are crucial for its biological activity, influencing its interaction with biological targets such as proteins and enzymes. The stereochemistry of (-)-quinine is essential for its therapeutic efficacy, particularly in its use as an antimalarial agent. The specific spatial arrangement of the substituents around the chiral centers determines the molecule’s overall three-dimensional shape, which is critical for its function and interaction within biological systems.
Case studies to illustrate the importance of chirality in natural products.
Paclitaxel (Taxol)
Taxol is derived from the Pacific yew tree, is a highly effective anticancer agent. The compound is chiral, and its anticancer activity is attributed to a specific enantiomer. Synthetic efforts have focused on isolating and producing the active enantiomer, as the inactive form does not possess the same therapeutic benefits.
Camptothecin
Camptothecin is another example, that is a potent anticancer natural product discovered in the bark and stem of the Camptotheca acuminata tree. The compound is chiral, and its derivatives, such as irinotecan and topotecan, are used as chemotherapy drugs. The chirality of these molecules plays a crucial role in their interactions with cellular targets, contributing to their pharmacological effects. (S)-Campothecin is found to be biologically active.
Artemisinin:
Artemisinin, a natural product extracted from the sweet wormwood plant (Artemisia annua), is highly effective against malaria, one of , the deadliest infectious diseases globally. Artemisinin contains several chiral centers, and its therapeutic activity is attributed to a specific enantiomer. Understanding and utilizing the correct enantiomer have been essential in producing effective antimalarial drugs.
Galantamine:
Galantamine is a natural product derived from the snowdrop plant (Galanthus spp.) and other members of the Amaryllidaceae family. It is used to treat Alzheimer’s disease. Galantamine is chiral, and the specific enantiomer responsible for the desired pharmacological effects is isolated and utilized in the drug formulation.
Ergotamine:
Ergotamine is a natural product produced by the ergot fungus (Claviceps purpurea). Historically, it has been used to treat migraines and has been the basis for the development of other antimigraine drugs. Ergotamine contains multiple chiral centers, and the pharmacological activity resides in specific enantiomers.
Chirality in Animals
In the animal kingdom, chirality manifests in various physiological and behavioral traits. A well-known example is the chirality observed in the shells of snails, which can be either left-handed (sinistral) or right-handed (dextral). This chirality is genetically determined and can influence mating behavior and reproductive success. In addition to physiological traits, chirality also affects animal behavior. Many animals, including humans, exhibit handedness, a preference for using one limb over the other. This behavioral chirality can impact foraging efficiency, tool use, and social interactions. The presence of chirality in both physiological and behavioral aspects underscores its evolutionary significance in animals
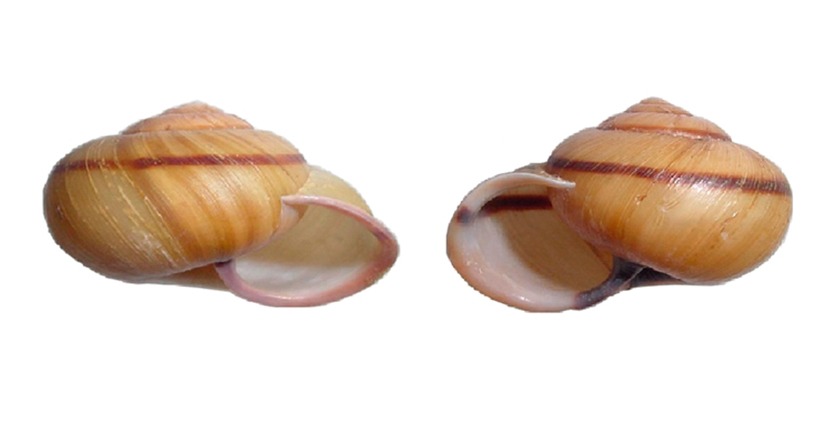
This image illustrates the concept of chirality through the comparison of two snail shells. The shells exhibit opposite coiling directions, with the left shell displaying a left-handed (sinistral) spiral and the right shell exhibiting a right-handed (dextral) spiral. This natural occurrence of chirality is a vivid example of how the same basic structure can have two non-superimposable mirror-image forms, similar to left and right hands. The chirality in snail shells, as in other biological structures, can influence their growth patterns and ecological interactions.
Natural Occurrences and Their Implications
Geological and Environmental Chirality
Chirality is not limited to biological systems; it also occurs in geological formations. Certain minerals exhibit chirality, with quartz being a notable example. Quartz crystals can exist in left-handed or right-handed forms, and this chirality can influence the optical properties of the mineral. Environmental factors, such as the Earth’s magnetic field and geochemical conditions, can affect the formation and distribution of chiral minerals. Understanding the chirality of minerals provides insights into geological processes and the history of the Earth’s formation.
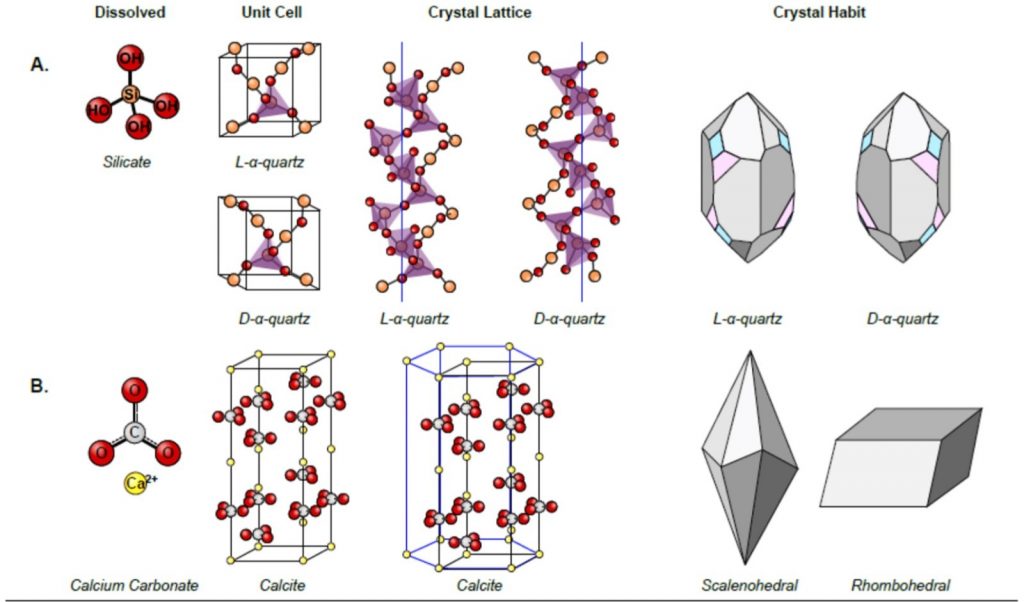
This figure illustrates chirality in crystalline structures, focusing on the differences between quartz and calcite. In section A, the chirality of quartz is depicted through its dissolved form, unit cell, crystal lattice, and crystal habit. The silicate ion forms the basis of quartz, and the unit cells of L-α-quartz and D-α-quartz are shown as mirror images. The crystal lattice structures further emphasize the opposite helical twists of L-α-quartz and D-α-quartz, and their external crystal shapes, or habits, highlight their non-superimposable mirror image forms, underscoring their chiral nature. In contrast, section B presents calcite, which does not exhibit chirality. The calcium carbonate molecule and its crystal lattice are shown, along with typical crystal habits such as scalenohedral and rhombohedral forms, demonstrating the achiral nature of calcite. This comparison vividly showcases how chirality is manifested in natural mineral structures, with quartz serving as a clear example of chirality and calcite illustrating an achiral structure.
Evolutionary Implications
The evolutionary implications of chirality are profound. Chirality can confer advantages in survival and reproduction, influencing the evolutionary trajectory of organisms. For example, the chirality of amino acids in proteins affects their structure and function, providing selective advantages to organisms with specific chiral configurations. In some cases, chirality can also play a role in reproductive isolation and speciation. The study of chirality in an evolutionary context sheds light on the mechanisms of natural selection and adaptation.
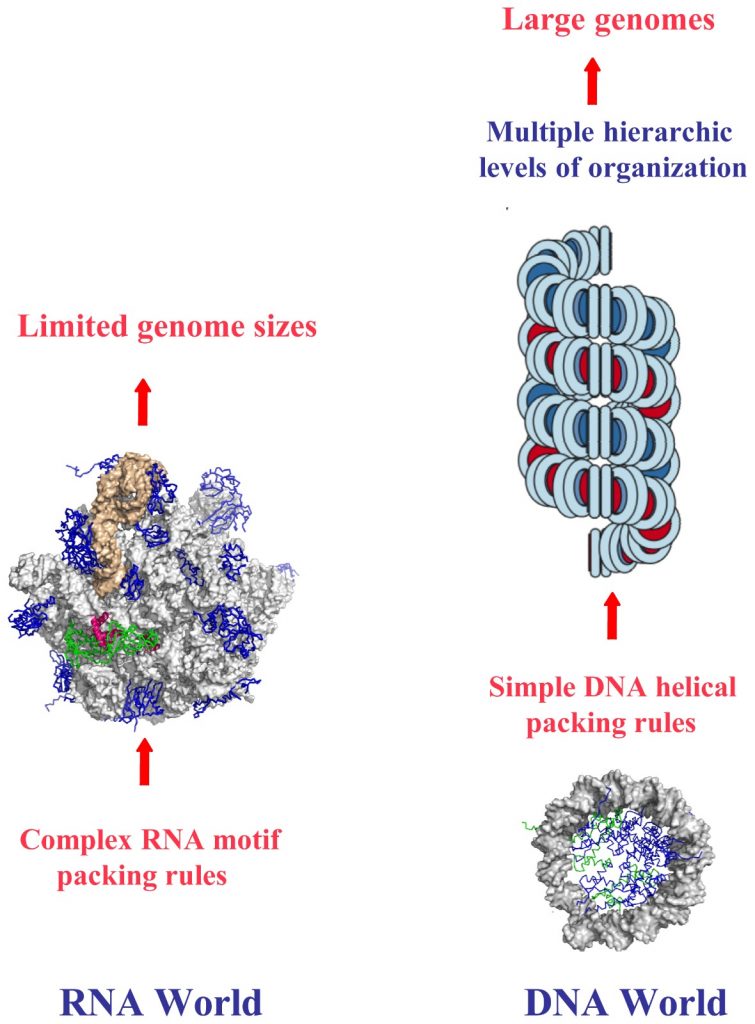
This figure contrasts the structural and organizational differences between RNA and DNA worlds, emphasizing the role of chirality in their hierarchical packing rules and genome sizes. In the RNA world (left), the complex RNA motif packing rules limit genome sizes due to the intricate folding and structural diversity of RNA molecules. The chirality of RNA contributes to these complex interactions, as the single-stranded nature of RNA allows for a variety of three-dimensional conformations, which are critical for its function but restrict the overall genome size.
In the DNA world (right), the simple helical packing rules of DNA enable the formation of large genomes. DNA’s double-helical structure, dictated by its right-handed chirality, allows for efficient and regular packing into chromatin and higher-order structures. This hierarchical organization, from the double helix to nucleosomes and chromatin fibers, supports the storage of vast amounts of genetic information. The chirality of DNA plays a crucial role in this process, as the uniformity and stability of the right-handed double helix facilitate the orderly and compact packaging necessary for managing large genomes. The figure illustrates how the inherent chirality of these nucleic acids influences their structural complexity and genomic capabilities.
Medical and Pharmaceutical Implications
Chirality has significant implications in medicine and pharmacology. Many natural toxins and venoms are chiral, with their biological activity dependent on their chirality. For instance, the chiral toxin tetrodotoxin, found in pufferfish, is a potent neurotoxin that affects sodium channels in nerve cells. Understanding the chirality of natural toxins is crucial for developing antidotes and therapeutic agents. In drug discovery, natural chiral compounds often serve as lead structures for developing new medications. The chirality of these compounds influences their interaction with biological targets, impacting their efficacy and safety. The study of chirality in natural products continues to drive advancements in pharmaceutical research and development.
Conclusion
Chirality is a fundamental property that shapes the structure and function of molecules in nature. From the double helix of DNA to the handedness of snail shells, chirality influences a wide range of biological systems and processes. Understanding chirality provides valuable insights into the molecular mechanisms that underpin life and offers opportunities for innovation in medicine, materials science, and environmental studies. As research into chirality advances, we can expect to uncover new applications and deepen our appreciation for the intricate symmetry and asymmetry that define the natural world.
Further Reading
Left-Handed DNA: Is That Right? https://www.promegaconnections.com/left-handed-dna-is-that-right
https://sandwalk.blogspot.com/2015/03/on-handedness-of-dna.html
https://cosmosmagazine.com/science/biology/is-there-asymmetry-in-nature
Noemie Globus and Roger D. Blandford, The Chiral Puzzle of Life, The Astrophysical Journal Letters, Volume 895, Number 1, 2020. DOI 10.3847/2041-8213/ab8dc6
Roger A. Hegstrom and Dilip K. Kondepudi, The Handedness of the Universe, Scientific American, January, 108-115, 1990.
https://biyokimya.vet/en-gb/the-importance-of-chirality-in-biological-systems/
Krastel, P., Petersen, F., Roggo, S., Schmitt, E., & Schuffenhauer, A. (2006). Aspects of Chirality in Natural Products Drug Discovery. Chirality in Drug Research, 67–94. doi:10.1002/9783527609437.ch3
Inaki M, Liu J, Matsuno K. 2016 Cell chirality: its origin and roles in left – right asymmetric development. Phil. Trans. R. Soc. B 371: 20150403. http://dx.doi.org/10.1098/rstb.2015.0403
https://www.the-scientist.com/cell-chirality-offers-clues-to-the-mystery-of-body-asymmetry-69584
https://chiralpedia.com/blog/quinine/
Ferdinand Devínsky, Chirality and the Origin of Life, Symmetry 2021, 13(12), 2277; https://doi.org/10.3390/sym13122277
Other Blogs on #ChiralityonFriday
1.Introduction to Chirality: Understanding the Basics
2. Molecular Handedness: How Chirality Shapes Molecules
3. Chirality in Nature: From DNA to Snail Shells
4. The Chemistry of Taste and Smell: How Chirality Affects Senses
5. Chiral Chemistry in Everyday Life: Hidden Handedness Around Us
7. https://chiralpedia.com/blog/chirality-in-materials-science-designing-with-handedness/
8. Chirality in Space: Cosmic Asymmetry and the Origins of Life
9. The Future of Chirality: Innovations and Emerging Trends
10. Chirality and You: Understanding and Appreciating Molecular Handedness