Lead
Chirality, or molecular handedness, is a fundamental concept that has far-reaching implications in various scientific fields. In materials science, chirality plays a crucial role in designing and developing advanced materials with unique properties and functionalities. From enhancing the performance of optical devices to improving the selectivity of catalysts, the application of chirality in materials science is both broad and significant. This blog explores the applications of chirality in materials science, focusing on chiral polymers and liquid crystals, and examines the impact of chirality on material properties and uses.
Applications of Chirality in Materials Science
Definition and Basic Concepts
Chirality in materials science refers to the property of materials that makes them non-superimposable on their mirror images. This characteristic is crucial in designing materials with specific optical, electronic, and mechanical properties. The unique handedness of chiral materials allows them to interact with light, electricity, and other materials in distinct ways, making them invaluable in various technological applications.
Overview of Applications in Various Sectors
Chirality finds applications in several sectors, including optical materials, electronics, photonics, catalysis, and biomedical applications. In optical materials, chiral compounds are used to create circularly polarized light, which is essential in advanced display technologies and optical communication systems. In electronics and photonics, chiral materials enhance the performance of devices by enabling more efficient light manipulation and signal processing. In catalysis, chiral catalysts are used to produce enantiomerically pure compounds, which are vital in pharmaceuticals and fine chemicals. In pharmaceutical and biomedical applications, chiral materials are employed for enantiomeric separation, in drug delivery systems, tissue engineering, and diagnostic devices, leveraging their unique interactions with biological molecules.
Chiral Interface
The concept of a chiral interface is a emerging concept in the study of chirality within materials science. This image encapsulates the multi-faceted roles that chiral interfaces play in various scientific applications. It highlights the interconnected areas of chiral recognition, separation, and catalytic reactions, showing how intrinsic chiral nanomaterials, chiral crystalline interfaces, and other specialized structures contribute to advancements in these fields.
The visualization also emphasizes the broader impact of chiral interfaces, illustrating their central position in driving innovations in drug development, materials engineering, and asymmetric catalysis. By understanding and leveraging these interfaces, scientists and engineers can design more efficient, selective, and effective materials and processes, ultimately pushing the boundaries of what is possible in materials science.

Bridging Science and Innovation
Chiral Interface, symbolizes the central role these interfaces play in chiral recognition, separation, and catalytic reactions. Surrounding this core are various applications and types of chiral interfaces:
Intrinsic Chiral Nanomaterials: These materials possess chirality inherently and are used in various applications due to their unique properties.
Chiral Crystalline Interfaces: These interfaces are structured at the molecular level to exhibit chirality, crucial for various recognition processes.
Chiral Molecule Modification: Involves the modification of molecules to impart chirality, enhancing their interaction with other chiral entities.
Chiral Molecular Imprinting: A technique used to create recognition sites for specific chiral molecules, aiding in their identification and separation.
Chiral Porous Framework: These frameworks are designed to selectively interact with chiral molecules, useful in separation and catalytic processes.
The outer ring highlights the broader categories of applications:
Chiral Recognition: The ability of chiral materials to distinguish between different enantiomers.
Chiral Separation: Techniques for separating chiral molecules based on their handedness.
Catalytic Reactions: Utilizing chiral interfaces to catalyze reactions that produce enantiomerically pure compounds.
Specific Applications
Chiral molecular sieves
The synthesis of a chiral molecular sieve has been a long-standing goal in the field of zeolites and molecular sieves because this type of inorganic material could open new avenues for asymmetric catalysis and chiral separations. The robust nature of these porous solids could show commercial advantages in chiral processes, as these materials are easily regenerated and thus used for many cycles of performance

Chiral Resolution Using Molecular Sieves
This image depicts the separation of enantiomers using chiral molecular sieves. The left side shows the S-enantiomer interacting with the S-molecular sieve, while the right side shows the R-enantiomer interacting with the R-molecular sieve. The mirror plane in the center highlights the chiral specificity of the sieves, which selectively adsorb one enantiomer over the other, facilitating chiral resolution.
Chiral membranes
Most pharmaceuticals are stereoisomers that each enantiomer shows dramatically different biological activity. Therefore, the production of enantiopure chemicals through sustainable and energy-efficient technology is one of the main objectives in the pharmaceutical industry. Membrane-based separation is a continuous process performed on a large scale that uses far less energy than the conventional thermal separation process.
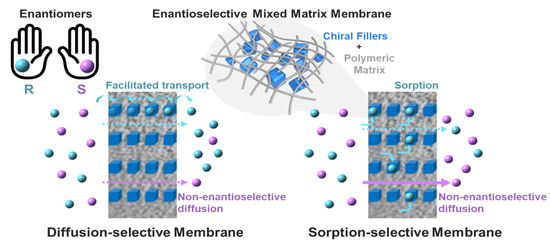
Principles between a diffusion – elective membrane and sorption – selective membrane.
Two mechanisms are suggested for chiral resolution using membrane-based processes: facilitated transport and retarded transport. Enantioselective membranes generally fall into two categories: ‘diffusion-selective’ and ‘sorption-selective,’ corresponding to the facilitated and retarded transport mechanisms, respectively. In diffusion-selective membranes, one enantiomer with a higher binding affinity predominantly interacts with the chiral active sites within the membrane. This interaction facilitates preferential adsorption from the feed stream and rapid transfer between chiral sites under a chemical potential gradient. Initially, this process can achieve an enantiomeric excess (ee) value close to 100% through selective permeation of one enantiomer. However, over time, the resolution efficiency diminishes due to the non-enantioselective diffusion of the other enantiomer.
Chiral nanosheets
Most porous 2D nanomaterials are achiral, and thus, their pores do not respond to chiral molecules. Chiral nanosheets are challenging to construct. However, such materials are desirable because they can be used for fast and efficient enantiomer separation.
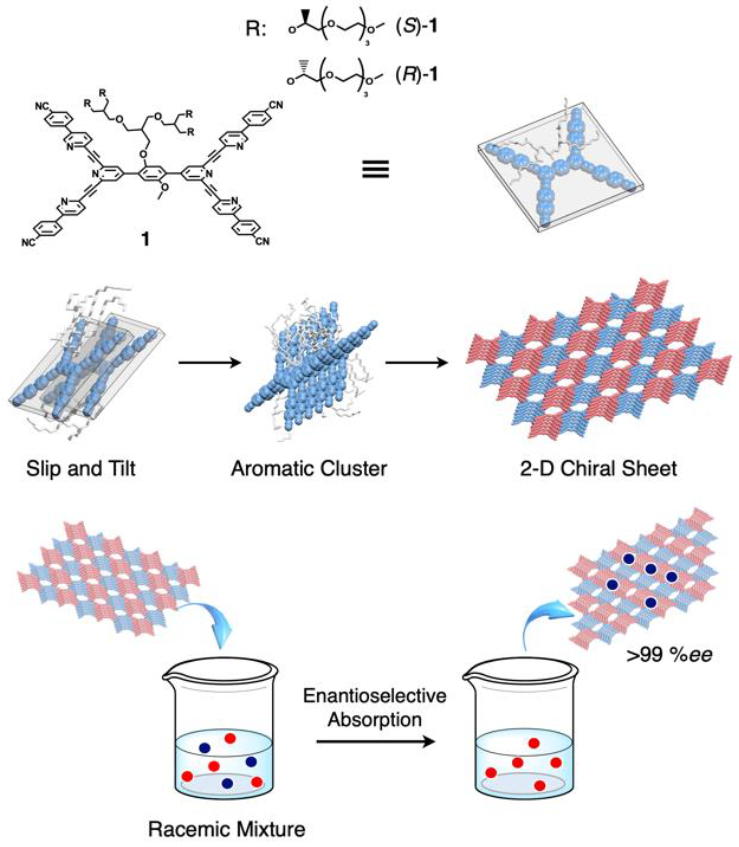
Source: Xiaopeng Feng, Bowen Shen, Bo Sun, Jehan Kim, Xin Liu, Myongsoo Lee,
Angew. Chem. Int. Ed. 2020.
https://doi.org/10.1002/anie.202003807
Being picky
(a) Molecular structure of 1S. (b) Graphical representation for the
formation of a single-layered chiral nanosheet structure through lateral
assembly of chiral clusters consisting of slipped planar aromatic segments. (c) Enantioselective absorption of the chiral sheets in a racemic mixture solution.
Single-layered chiral 2D sheet structures with dual chiral void spaces form in aqueous methanol solution from discrete clusters of planar aromatic segments arranged in an in-plane up and down AB packing. The slipped cofacial stacking of these aromatic segments imparts chirality to the sheets. These nanosheets rapidly absorb one enantiomer from a racemic mixture solution with an enantiomeric excess (ee) greater than 99%.
Chiral Sensors and Detectors: Chiral materials are used in sensors and detectors to enhance their sensitivity and selectivity. For instance, chiral sensors can distinguish between different enantiomers of a compound, making them valuable in pharmaceutical analysis and environmental monitoring.
Enantioselective Catalysts: Chiral catalysts are crucial in chemical synthesis, particularly in the production of enantiomerically pure compounds. These catalysts facilitate reactions that produce one enantiomer preferentially over the other, ensuring the desired therapeutic effects and reducing side effects in pharmaceuticals.
Chiral Polymers and Liquid Crystals
Chiral Polymers
Chiral polymers are polymers that possess chirality in their backbone or side chains. These materials are synthesized using chiral monomers or through asymmetric polymerization processes. Chiral polymers have diverse applications, particularly in the biomedical field. For example, they are used in drug delivery systems to enhance the targeted delivery and release of therapeutics. In tissue engineering, chiral polymers provide scaffolds that mimic the natural chiral environment of biological tissues, promoting cell growth and differentiation. Additionally, biodegradable chiral polymers are developed for environmentally friendly packaging and medical
Examples and Case Studies: Polylactic Acid (PLA): PLA is a biodegradable polymer synthesized from lactic acid, which exists in two enantiomeric forms. The properties of PLA can be tailored by controlling the ratio of these enantiomers, making it suitable for applications ranging from medical implants to eco-friendly packaging devices.
Chiral Liquid Crystals
Chiral liquid crystals are materials that combine the fluidity of liquids with the ordered structure of crystals. These materials exhibit unique optical properties, such as the ability to rotate polarized light, which makes them ideal for use in display technologies and optical devices. Chiral liquid crystals are used in liquid crystal displays (LCDs) to improve the quality and efficiency of the display. They are also employed in tunable optical filters and photonic devices, where their ability to manipulate light enhances device performance.
Examples and Case Studies: Cholesteric Liquid Crystals: Cholesteric liquid crystals, a type of chiral liquid crystal, are used in reflective displays and tunable lasers. Their ability to selectively reflect light of specific wavelengths makes them valuable in creating high-resolution, color-tunable displays.
Impact of Chirality on Material Properties and Uses
Effect on Physical Properties
Chirality significantly affects the physical properties of materials, including their mechanical, optical, and electrical properties. For instance, chiral materials can exhibit unique mechanical strength and flexibility, making them suitable for applications that require durable and adaptable materials. In optics, chiral materials can manipulate light in ways that achiral materials cannot, enabling the development of advanced optical devices with enhanced performance. Electrically, chirality can influence the conductivity and charge transport properties of materials, impacting their use in electronic devices.
Influence on Material Functionality
The unique properties of chiral materials enhance their functionality in specific applications. For example, chiral polymers used in drug delivery systems can improve the targeting and release profiles of therapeutics, enhancing their efficacy and reducing side effects. In catalysis, chiral catalysts improve the selectivity and efficiency of chemical reactions, leading to higher yields of desired products. The ability of chiral materials to interact selectively with other molecules and their environment makes them indispensable in advanced material design.
Case Studies: Chiral Photonic Crystals: These materials exhibit unique optical properties that make them suitable for use in photonic devices, such as optical fibers and waveguides. Their ability to control the flow of light enhances the performance and efficiency of these devices.
Challenges and Future Prospects
Despite the significant advantages of chiral materials, there are challenges associated with their synthesis and application. Achieving high enantiomeric purity and controlling the chirality of materials can be technically demanding and costly. However, advancements in synthesis techniques, such as asymmetric synthesis and biocatalysis, are addressing these challenges. Future research is likely to focus on developing more efficient and sustainable methods for producing chiral materials, exploring new applications, and understanding the fundamental principles that govern their behavior.
Conclusion
Chirality is a fundamental aspect of materials science that influences the design and functionality of advanced materials. From chiral polymers and liquid crystals to enantioselective catalysts and sensors, chirality plays a crucial role in enhancing the performance and efficiency of materials in various applications. As research continues to advance, the potential for chirality in materials science is vast, promising new discoveries and innovations that will drive progress in technology and industry. Understanding and leveraging the unique properties of chiral materials will be key to developing the next generation of high-performance materials.
Further Reading
Molecular Sieve: Purpose, Origin, How Is It Made, Types, and Uses | Xometry
New Porous Solids May Lead to Better Drugs – www.caltech.edu
Advanced Information – The Nobel Prize in Chemistry 2001
Qixin Liu, Yuqi Zhang, Lie WU, Xiue Jiang, Recent progress in fabrication and application of chiral interfaces, Chinese Journal of Analytical Chemistry, 2024. https://doi.org/10.1016/j.cjac.2024.100391
Choi, H.-J.; Ahn, Y.-H.; Koh, D.-Y. Enantioselective Mixed Matrix Membranes for Chiral Resolution. Membranes 2021, 11, 279. https://doi.org/10.3390/membranes11040279
Rui Xie et al., Membranes and membrane processes for chiral resolution, Chem. Soc. Rev., 2008, 37, 1243–1263 | DOI: 10.1039/b713350b. https://pubs.rsc.org/en/content/articlelanding/2008/cs/b713350b
MacKenzie, L.E., Stachelek, P. The twists and turns of chiral chemistry. Nat. Chem. 2021, 13, 521–522. https://doi.org/10.1038/s41557-021-00729-8
Xiaopeng Feng, Bowen Shen, Bo Sun, Jehan Kim, Xin Liu, Myongsoo Lee, Angew. Chem. Int. Ed. 2020. https://doi.org/10.1002/anie.202003807
Ham, S.-H.; Han, M.J.; Kim, M. Chiral Materials for Optics and Electronics: Ready to Rise? Micromachines 2024, 15, 528. https://doi.org/10.3390/mi15040528
Zor, E., Bingol, H., & Ersoz, M. (2019). Chiral Sensors. TrAC Trends in Analytical Chemistry, 115662. doi:10.1016/j.trac.2019.1156
Other Blogs on #ChiralityonFriday
1. Introduction to Chirality: Understanding the Basics
2. Molecular Handedness: How Chirality Shapes Molecules
3. Chirality in Nature: From DNA to Snail Shells
4. The Chemistry of Taste and Smell: How Chirality Affects Senses
5. Chiral Chemistry in Everyday Life: Hidden Handedness Around Us
6. Chirality in Pharmaceuticals: The Impact of Molecular Handedness on Medicine
7. https://chiralpedia.com/blog/chirality-in-materials-science-designing-with-handedness/
8. Chirality in Space: Cosmic Asymmetry and the Origins of Life
9. The Future of Chirality: Innovations and Emerging Trends
10. Chirality and You: Understanding and Appreciating Molecular Handedness