Introduction
Asymmetric synthesis has made significant strides in recent decades, becoming a crucial component of pharmaceutical, agrochemical, and fine chemical industries. The development of chiral catalysts and the understanding of stereoselective reactions have enabled the production of enantiomerically pure compounds on an industrial scale. This blog explores the future perspectives in chiral asymmetric synthesis, focusing on emerging technologies, interdisciplinary approaches, global impact, and the challenges that lie ahead.
Emerging Technologies
Green Chemistry Approaches
The future of chiral asymmetric synthesis is increasingly aligned with the twelve principles of green chemistry. Sustainable catalytic systems are being developed to minimize the environmental footprint of chemical processes.
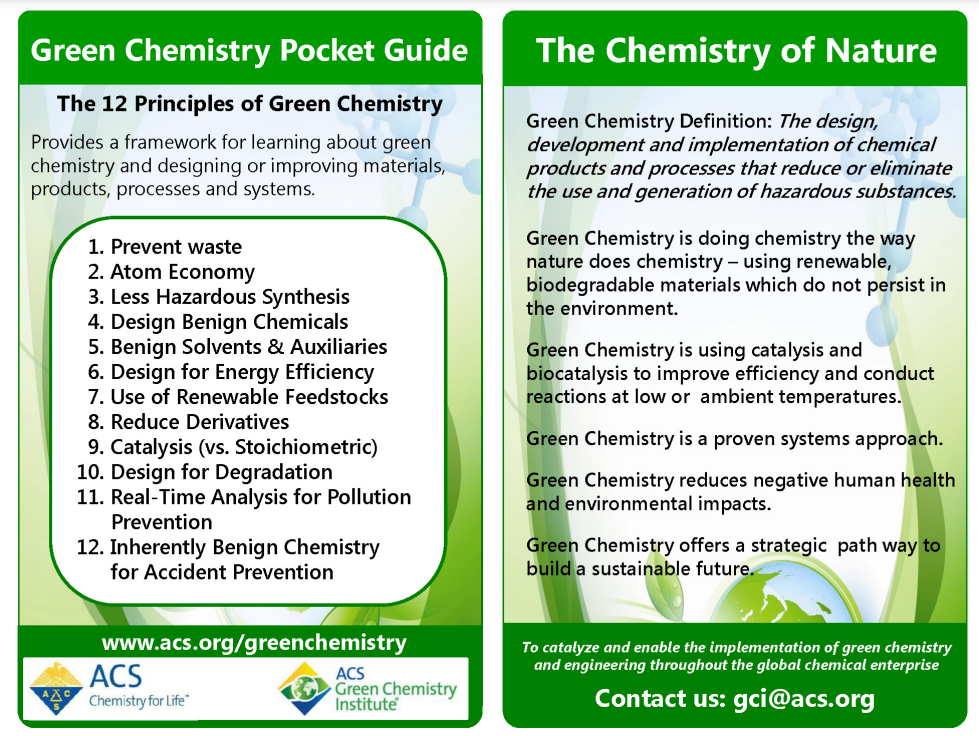
The necessity of more sustainable conditions that follow the principles of Green Chemistry have pushed researchers to the development of novel reagents, catalysts and solvents for greener asymmetric methodologies. These include:
- Atom Economy:
Atom economy is all about designing chemical reactions in a way that ensures almost all the reactants end up in the final product, which helps reduce waste. When we talk about synthetic methods, the goal is to make sure that all the materials used in the process are incorporated into the final product. Atom Economy, which is the second principle of green chemistry, focuses on minimizing waste at the molecular level. It’s a green chemistry metric that helps us gauge how efficient a reaction is. The equation for atom economy essentially tells us the percentage of atoms from the reactants that make it into the final product. The higher the atom economy, the better, because any atoms that don’t end up in the final product are considered wasted.
- Biodegradable Catalysts: Catalysts that can be easily broken down into non-toxic components after use, reducing environmental contamination.
- Organocatalysts:
Creating new drugs involves a key step: synthesizing the drug candidates, their important intermediates, and the active pharmaceutical ingredients (APIs). This process aims to find methods that are highly effective, yield good results, and are environmentally friendly. Additionally, making APIs on a large scale requires careful analysis to ensure the use of less toxic chemicals, greener solvents, more efficient techniques, and reduced waste, all of which are crucial for sustainable production.
In the world of asymmetric catalysis, there are three main approaches: biocatalysis, metal catalysis, and organocatalysis. Biological catalysts, like isolated enzymes or whole cells, are increasingly used in industrial settings, especially for hydrolytic reactions. The rapid advancements in organometallic chemistry and catalysis have significantly contributed to organic synthesis. However, the extensive use of metal compounds has brought attention to environmental and toxicity concerns.
In recent years, the chemical science and pharmaceutical industries have been moving away from using catalytic metal complexes and instead embracing organocatalysts. These small chiral organic molecules have proven to be highly effective and versatile, enriching the field of organic synthesis. Some examples include chiral proline derivatives, N-heterocyclic carbenes, chiral thioureas, Brønsted acids, and phase-transfer catalysts like quaternary ammonium salts derived from cinchona alkaloids.
Additionally, continuous flow chemistry has emerged as a promising approach for green and sustainable synthesis. It offers exceptional opportunities to speed up, integrate, simplify, scale up, and automate chemical reactions. Earlier this year, Ötvös and Kappe reviewed various flow chemistry-based methods for synthesizing chiral active pharmaceutical ingredients (APIs) and their advanced stereogenic intermediates, highlighting the use of biocatalysis, organometallic catalysis, and organocatalysis.
Case Study: Greener Asymmetric Synthesis of Antiviral Drugs via Organocatalysis
Many antiviral agents used in clinical practice are amino acids, short peptides, or peptidomimetics. The table below showcases selected chiral antiviral drugs, with anti-HIV agents being prominent examples. These drugs often rely on asymmetric synthesis as a crucial step in their production. Most synthetic routes use chiral pools, such as amino acids, as starting materials. Chiral resolution using enzymes or metal catalysts has also been successfully employed, while enantioselective organocatalyzed methods have been used in a few cases. For a more detailed discussion, refer to the source article <Pharmaceuticals 2021, https://doi.org/10.3390/ph14111125>.
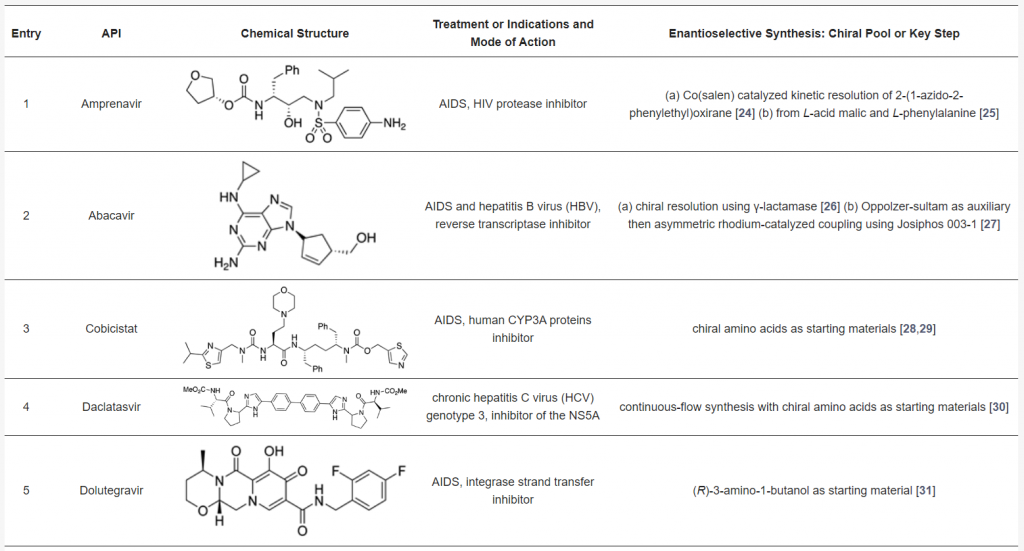
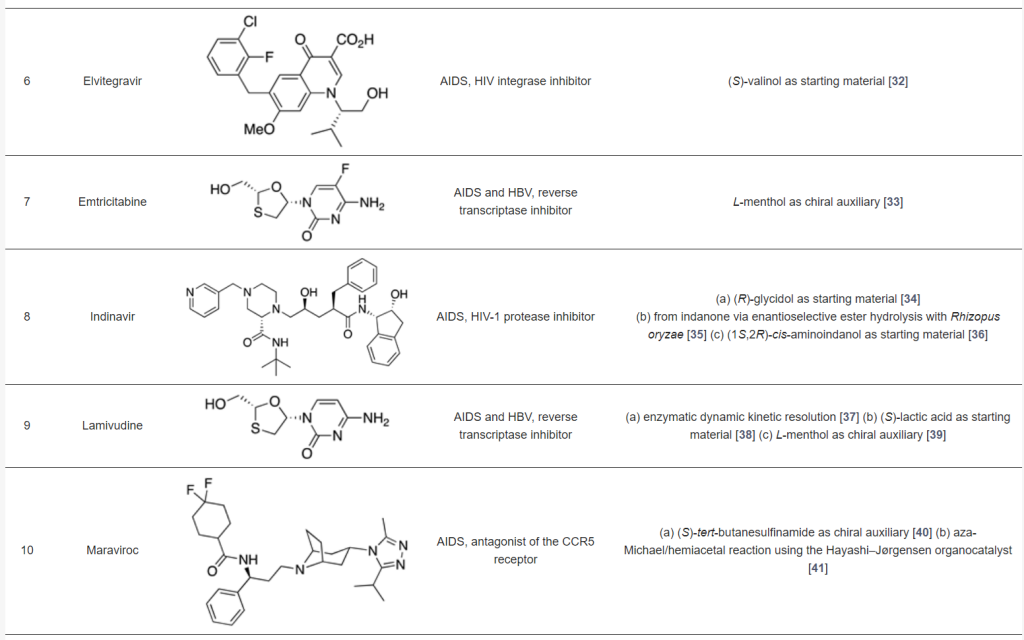
Chiral Antiviral Drugs
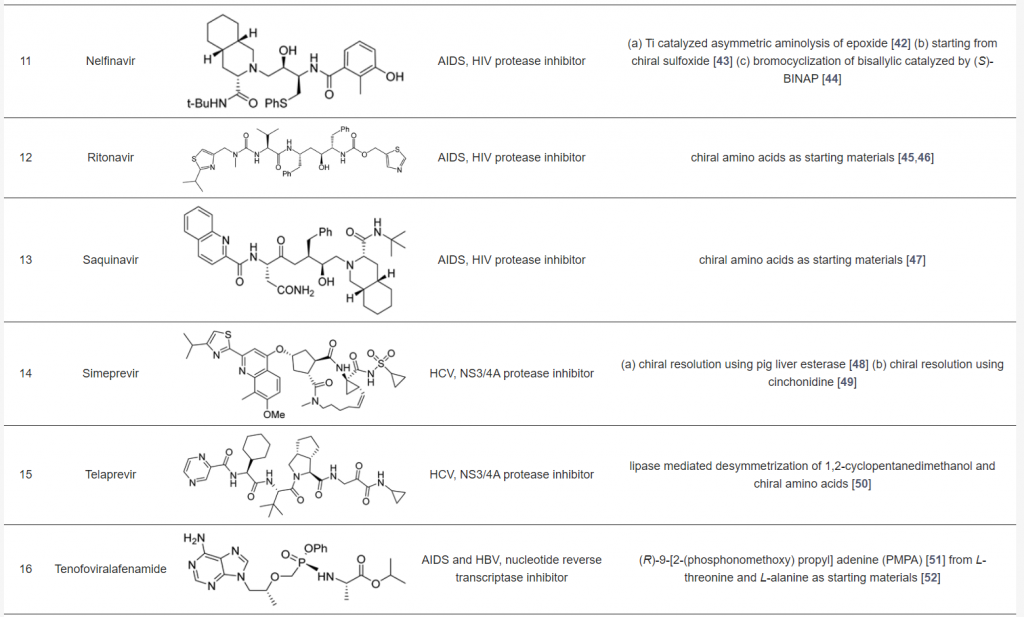
Chiral Antiviral Drugs
Photoredox Catalysis with Organocatalysis: Merger
Photoredox catalysis and organocatalysis are two influential methods for activating molecules, widely used in inorganic and organic chemistry. We combined these catalytic approaches to address challenges in asymmetric chemical synthesis.

Photoredox Dual Catalysis:
A Fertile Playground for the Discovery of New Reactivities
Photoredox catalysis and organocatalysis are two exciting methods used to activate molecules. They are widely used in inorganic and organic chemistry. The two approaches have been combined to solve challenges in asymmetric synthesis
Sustainable Catalytic Systems
Efforts are being made to develop catalytic systems that are not only efficient but also sustainable. Examples include:
- Bio-based Catalysts: Using renewable biological materials as catalysts, which can be sourced sustainably.
The development of new and more efficient catalytic systems is the subject of increasing attention from both academic and industrial research. Moreover, biomass is an endless supply of hydrocarbon materials that can be used as renewable raw materials for the development of new organic compounds. In this context, many research groups have devoted their works to products with a natural chirality source, non-toxic, biodegradable and usually cost effective for the development of new chiral catalysts. Some new chiral molecules derived from biomass such as carbohydrates and natural amino acids have been synthesized and used as chiral ligands or organocatalysts for asymmetric transformations leading to the formation of expected products in good yields with high enantioselectivities. However, the design and synthesis of novel chiral catalysts starting from biomass still presents a challenge and an opportunity to researchers.
- Recyclable Catalysts: Designing catalysts that can be easily recovered and reused without significant loss of activity or selectivity.
Current chiral catalysts encounter challenges pertaining to their separation from products and the recycling process. The utilization of chiral recyclable catalysts not only reduces production costs but also aligns with the growing emphasis on environmentally-friendly chiral synthetic chemistry. These recyclable catalysts exhibit diverse carriers and distinct characteristics. Chemists employ the distinctive attributes of individual carriers to render them recyclable, thereby yielding time and cost savings.
- Bio-based solvents for asymmetric organocatalytic processes
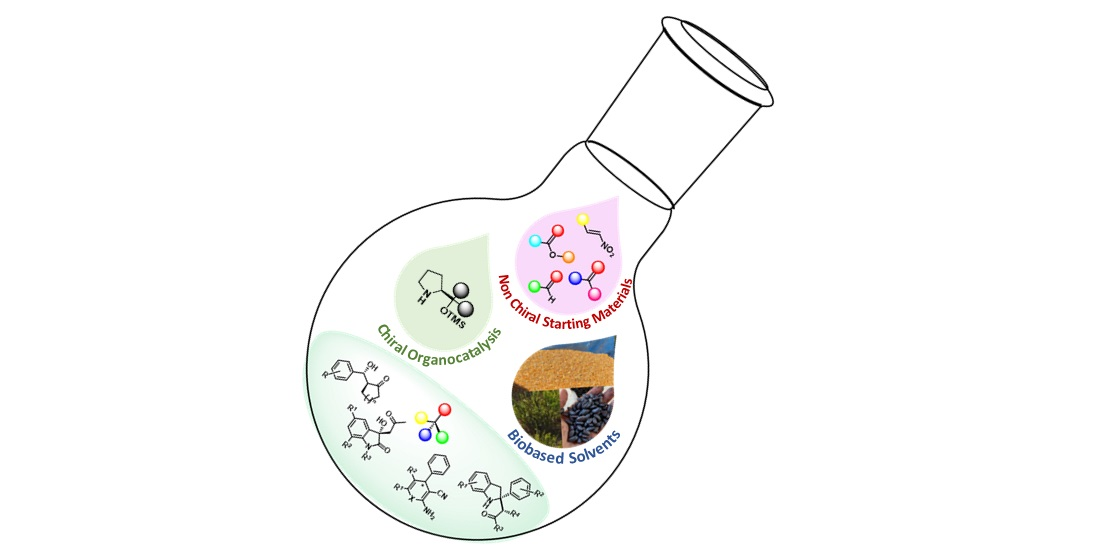
Bio-based solvents
Derived from vegetable, animal, or mineral raw materials using chemical and physical processes that are, in general, safer for the environment and humans.
Green Chemistry Innovation
A new green chemistry metric called the global E factor (EG factor) has been proposed. This metric not only considers the efficiency of the organocatalytic process but also the synthetic route to create the catalyst itself. Organocatalyst scaffolds are classified into four categories based on the system by List and Maruoka: Lewis bases, Brønsted bases, Brønsted acids, and Lewis acids. By classifying these scaffolds according to their E factor, we can get a reliable idea of the environmental impact of the synthesis process for each catalyst. The E factor is considered the most comprehensive mass-based parameter for evaluating the overall greenness of a process, especially from an industrial perspective.

Global E factor, EG
New green chemistry metric
Interdisciplinary Approaches
Integration with Synthetic Biology
Synthetic biology offers exciting possibilities for chiral asymmetric synthesis. By engineering microorganisms to produce chiral compounds, scientists can harness the specificity and efficiency of natural biosynthetic pathways. This approach can lead to the development of new biocatalysts and the production of chiral molecules under mild, environmentally friendly conditions.

Biocatalytic asymmetric synthesis
Low molecular weight synthetic peptides have proven to be effective catalysts for a growing range of asymmetric transformations. These peptide-based catalysts often enable new multifunctional substrate activation modes and achieve unprecedented levels of selectivity. Their ease of preparation, modular and adjustable structures, and often biomimetic characteristics make peptides excellent chiral catalysts and of great interest. Numerous examples of peptide-catalyzed asymmetric reactions have been documented in the literature.
Case Study: Biocatalytic of asymmetric synthesis
Synthetic biology can help develop chemicals with multiple chiral centers. Sitagliptin (Januvia) is a popular diabetes medication that works by inhibiting the DPP-4 enzyme, which in turn reduces the breakdown of GLP-1, leading to increased insulin secretion. By 2021, Januvia’s market reached $1.4 billion. Traditionally, the chemical synthesis of sitagliptin involved using a rhodium-based chiral catalyst, which had low stereoselectivity and resulted in rhodium contamination in the product. However, a new approach using transaminase and synthetic biology, including techniques like homologous modeling and saturation mutagenesis, has significantly improved the efficiency and purity of sitagliptin synthesis. For more details, you can refer to the article in Science, 2010, 329, 305. doi: 10.1126/science.1188934.
Role of Chemoinformatics and machine-learning techniques in organocatalysis
A significant revolution is on the horizon that could transform computer-assisted catalyst design. Until now, computational techniques like Hybrid Quantum Mechanics/Molecular Mechanics (QM/MM) and Density Functional Theory (DFT) have been invaluable in catalysis. They provide insights into reaction mechanisms and occasionally help fine-tune catalysts based on stereochemical models. However, these methods haven’t been very effective at predicting outcomes. Catalyst design has largely relied on human creativity, with fine-tuning often being a tedious, trial-and-error process that doesn’t always yield optimal results due to the limited number of candidates tested.
Today, artificial intelligence (AI) and machine learning (ML) are starting to change this landscape. With enhanced computing power, more data, and advanced algorithms, AI is becoming a valuable tool in chemistry. AI and ML are revolutionizing asymmetric synthesis by analyzing large datasets of reaction outcomes. These algorithms can predict the enantioselectivity and efficiency of new catalysts, speeding up the discovery of novel chiral catalysts and optimizing existing processes. Future applications include:
- Predictive Modeling: Using AI to model complex catalytic systems and predict the outcomes of asymmetric reactions.
- Automated Synthesis Planning: Employing ML to design and execute synthetic routes for chiral compounds, reducing the need for trial-and-error experimentation.
Impact of Machine Learning
Global
In recent years, the use of machine learning (ML), including deep learning (DL), has grown rapidly, driving data-driven discoveries in various fields, especially bioinformatics and cheminformatics. ML uncovers hidden patterns in large datasets of biological and chemical information to make predictions or decisions. ML-based frameworks have been successfully applied to bioinformatics areas like predicting protein structures, identifying binding sites for protein-protein interactions, and discovering new therapeutic targets. Additionally, ML is widely used in cheminformatics to improve drug design efficiency, determine the best synthesis pathways for compounds, and predict the physicochemical properties of key molecules.
A study shows that research papers on ML applications in bio-cheminformatics come from countries such as the United States, China, Italy, Belgium, Brazil, India, Japan, South Korea, and the United Kingdom. These papers are believed to represent the global advancements in ML within bio-cheminformatics.
Asymmetric Synthesis in Developing Countries
The benefits of asymmetric synthesis extend globally, particularly in developing countries. By enabling the production of essential pharmaceuticals and agrochemicals locally, asymmetric synthesis can improve healthcare and agricultural productivity. Efforts to transfer technology and knowledge to these regions are crucial for fostering sustainable development.
Contribution to Global Health and Sustainability
Asymmetric synthesis plays a vital role in global health by facilitating the production of enantiomerically pure drugs, which are often more effective and have fewer side effects. Additionally, the development of environmentally friendly synthesis methods contributes to sustainability by reducing the chemical industry’s ecological impact.
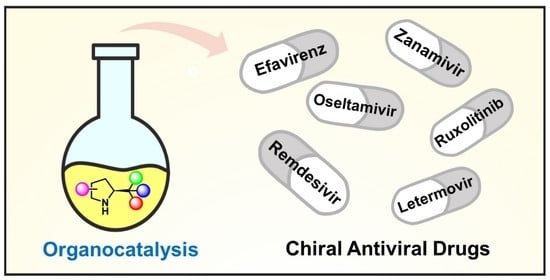
Greener Asymmetric Synthesis of Antiviral Drugs via Organocatalysis
Viral infections lead to many serious human diseases and high mortality rates. Consequently, both academic researchers and the pharmaceutical industry are constantly on the lookout for new antiviral compounds. They also face the challenge of creating greener and more efficient synthesis methods. This is particularly crucial for drugs with stereogenic centers, which need highly enantioselective processes. To address this, organocatalytic methods, a greener approach to asymmetric synthesis, are being used to enhance the efficiency and sustainability of synthetic routes for important commercial antiviral chiral drugs.
Future Challenges
Addressing Remaining Technical Barriers
Despite the progress made, several technical barriers remain:
- Scalability: Ensuring that laboratory-scale asymmetric synthesis can be scaled up efficiently for industrial production.
- Selectivity: Achieving high enantioselectivity for a broader range of substrates remains a challenge.
- Cost: Reducing the cost of chiral catalysts and synthesis processes to make them economically viable for widespread use.
Ensuring Economic Viability
For asymmetric synthesis to be widely adopted, it must be economically viable. This involves:
- Cost-Effective Catalysts: Developing catalysts that are affordable and effective.
- Process Optimization: Streamlining synthetic processes to reduce costs and improve efficiency.
Conclusion
The future of chiral asymmetric synthesis is bright, with numerous opportunities for innovation and interdisciplinary collaboration. By embracing green chemistry principles, integrating synthetic biology, and leveraging AI and machine learning, the field can overcome current challenges and achieve new levels of efficiency and sustainability. Creativity has always been the engine of innovation. When combined with emerging technologies like electrochemistry, photocatalysis, and chemoinformatics, it is expected to significantly advance the field of organocatalysis in the coming years.
Encouraging innovation and collaboration across disciplines will be key to realizing the full potential of asymmetric synthesis. As researchers and industries work together, the advancements in this field will continue to contribute to global health, sustainability, and economic development, making a lasting impact on society.
The vision for the future of chiral asymmetric synthesis includes a commitment to sustainability, the use of cutting-edge technologies, and a focus on global collaboration. By pursuing these goals, the field will not only advance scientifically but also provide tangible benefits to people around the world.
Further Reading
Anastas, P. T.; Warner, J. C. Green Chemistry: Theory and Practice, Oxford University Press: New York, 1998, p.30.
Giorgianni, G.; Bernardi, L.; Fini, F.; Pesciaioli, F.; Secci, F.; Carlone, A. Asymmetric Organocatalysis—A Powerful Technology Platform for Academia and Industry: Pregabalin as a Case Study. Catalysts 2022, 12, 912. https://doi.org/10.3390/catal12080912
Thi Thuy Duong Ngo, et al., Chiral catalysts derived from biomass: design, synthesis and applications in asymmetric catalysis, Vietnam J. Chem., 2019, 57(6), 670-680. DOI: vjch.201900177
A. Antenucci, S. Dughera, P. Renzi, Green Chemistry Meets Asymmetric Organocatalysis: A Critical Overview on Catalysts Synthesis, ChemSusChem 2021, 14, 2785-2853. https://doi.org/10.1002/cssc.202100573.
da Silva, E.M.; Vidal, H.D.A.; Corrêa, A.G. Advances on Greener Asymmetric Synthesis of Antiviral Drugs via Organocatalysis. Pharmaceuticals 2021, 14, 1125. https://doi.org/10.3390/ph14111125.
Dr. Achille Antenucci, et al., Green Chemistry Meets Asymmetric Organocatalysis: A Critical Overview on Catalysts Synthesis, 2021, https://doi.org/10.1002/cssc.202100573.
Miele, M.; Pillari, V.; Pace, V.; Alcántara, A.R.; de Gonzalo, G. Application of Biobased Solvents in Asymmetric Catalysis. Molecules 2022, 27, 6701. https://doi.org/10.3390/molecules27196701.
Martelli, L.S.R.; Machado, I.V.; dos Santos, J.R.N.; Corrêa, A.G. Recent Advances in Greener Asymmetric Organocatalysis Using Bio-Based Solvents. Catalysts 2023, 13, 553. https://doi.org/10.3390/catal13030553.
Marco M. Mastandrea, Miquel A. Pericàs, Photoredox Dual Catalysis: A Fertile Playground for the Discovery of New Reactivities, European Journal of Inorganic Chemistry, 2021, https://doi.org/10.1002/ejic.202100455
GuiPing Han, Application of chiral recyclable catalysts in asymmetric catalysis, RSC Adv., 2024, 14, 16520-16545. doi: 10.1039/D4RA01050G.
Sanchez-Fuente, M., et al., Chiral Porous Organic Frameworks: Synthesis, Chiroptical Properties, and Asymmetric Organocatalytic Applications. Catalysts 2023, 13, 1042. https://doi.org/10.3390/catal13071042.
Molnár, Á., & Papp, A. Catalyst recycling – A survey of recent progress and current status. Coordination Chemistry Reviews, 2017, 349, 1–65. doi:10.1016/j.ccr.2017.08.01 https://doi.org/10.1016/j.ccr.2017.08.011.
Barry M. Trost, Asymmetric catalysis: An enabling science, PNAS, 2004, 101 (15) 5348-5355. https://doi.org/10.1073/pnas.0306715101.
Metrano AJ, Chinn AJ, Shugrue CR, Stone EA, Kim B, Miller SJ. Asymmetric Catalysis Mediated by Synthetic Peptides, Version 2.0: Expansion of Scope and Mechanisms. Chem Rev. 2020, 20,11479-11615. doi: 10.1021/acs.chemrev.0c00523.
Lassaletta, J.M. Spotting trends in organocatalysis for the next decade. Nat Commun, 2020, 11, 3787. https://doi.org/10.1038/s41467-020-17600-y
https://pubs.acs.org/doi/10.1021/acs.jcim.4c00444.
Lassaletta JM. Spotting trends in organocatalysis for the next decade. Nat Commun., 2020,11,3787. doi: 10.1038/s41467-020-17600-y.
Yan, X., Liu, X., Zhao, C. et al. Applications of synthetic biology in medical and pharmaceutical fields. Sig Transduct Target Ther., 2023, 8, 199. https://doi.org/10.1038/s41392-023-01440-5.
Christopher K. Savile et al., Biocatalytic Asymmetric Synthesis of Chiral Amines from Ketones: Applied to Sitagliptin Manufacture,
Science, 2010, 329, 305. doi: 10.1126/science.1188934.
Editorial: Machine Learning in Bio-cheminformatic, J. Chem. Inf. Model. 2024, 64, 7, 2125–2128. https://doi.org/10.1021/acs.jcim.4c00444.
Well structured article. One interesting element of using synbio process for production is the multiple benefits it offers – Reduced carbon footprint, reduced water footprint, Minimalized Field-to-Yield Ratios, Lesser stress on land used for cultivation, possibility of using renewable energy sources for powering the production fermenters, ability to divert organic waste matter as a carbon source for microorganisms, ability to customize the final products for its commercially favoured attributes – and hence the carbon credits earned will be quite significant.
Thank you for your insightful comment! I’m glad you found the article well-structured. The multiple benefits of synthetic biology (SynBio) in production, such as reduced carbon and water footprints, minimized field-to-yield ratios, and the use of renewable energy and organic waste, truly highlight its sustainability. Customizing final products for commercial attributes and earning significant carbon credits further showcase SynBio’s potential to revolutionize production and contribute to a sustainable future.