Abstract
Chiral pharmacology, which studies how different enantiomers (mirror-image forms of molecules) affect the body, has truly changed the landscape of pharmaceutical science. It highlights the importance of stereochemistry – the three-dimensional structure of molecules – in making drugs safer and more effective. Today, over half of modern medicines are chiral, but in the past, many were given as racemic mixtures (a blend of enantiomers), leading to unpredictable results.
This article delves into the fascinating world of chiral pharmacology, sharing key concepts like eutomer (the active enantiomer), distomer (the less active one), and eudismic ratio (how they compare in effect). It also touches on the historical breakthroughs, technical hurdles, and ethical considerations in this field, showing how enantiomer-specific drug development is reshaping medicine and advancing precision treatments.
Introduction to Chirality
Chirality, derived from the Greek cheir (hand), refers to molecules existing as non-superimposable mirror images (enantiomers). These enantiomers, though chemically identical in an achiral environment, interact differently with chiral biomolecules like enzymes and receptors. This dichotomy can lead to divergent therapeutic and toxicological outcomes (Ariëns, 1984). Understanding stereochemical terminology – such as the eutomer (active enantiomer) and distomer (inactive/harmful enantiomer) – is essential for grasping the nuances of drug action and optimization.
Historical Context: The Thalidomide Tragedy
The thalidomide disaster (1957–1961) underscored the importance of chirality. Marketed as a racemic sedative, its R-enantiomer alleviated morning sickness, while the S-enantiomer caused severe teratogenicity, resulting in over 10,000 birth defects (Blaschke et al., 1980). This catastrophe prompted global regulatory reforms, including the FDA’s 1992 policy mandating enantiomer-specific evaluations (FDA, 1992). Caldwell (1995) highlighted the pharmaceutical industry’s initial resistance to these reforms due to increased costs and technical challenges.
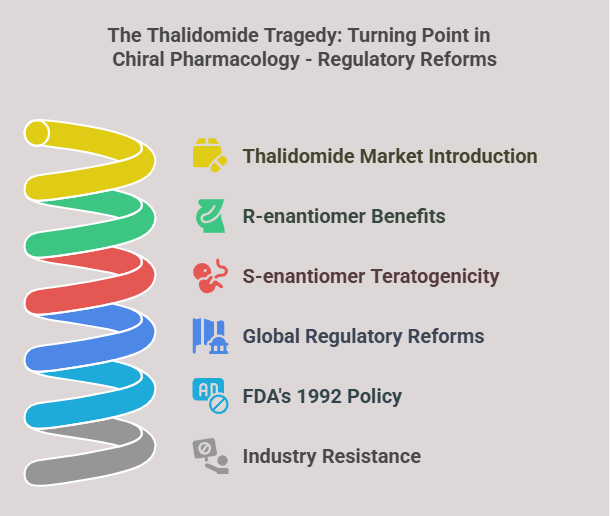
Scientific Principles of Chiral Pharmacology
Enantiomers, Eutomers, and Distomers
Enantiomers exhibit stereoselectivity in pharmacokinetics (PK) and pharmacodynamics (PD). The enantiomer with therapeutic activity is termed the eutomer, while its mirror image, often less active or toxic, is the distomer. The eudismic ratio quantifies their potency difference, guiding drug optimization (Lehmann et al., 1976). For example, the S-warfarin eutomer is 3–5 times more potent than the R-distomer due to enhanced binding to vitamin K epoxide reductase (Kaminsky & Zhang, 1997).
Metabolism and Toxicity
Cytochrome P450 enzymes metabolize enantiomers differentially. Escitalopram (S-citalopram), the eutomer, is metabolized slower than the R-distomer, prolonging its antidepressant effect (Owens et al., 2001). Conversely, the R-methadone distomer contributes to cardiotoxicity via QT prolongation (Eap et al., 2002).
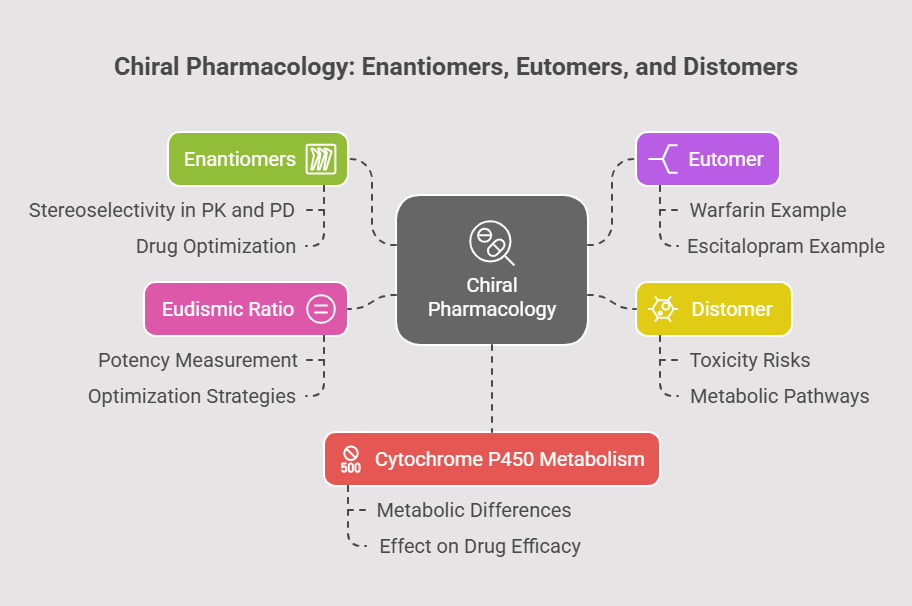
Challenges in Drug Development
Racemic vs. Single-Enantiomer Drugs
Racemic mixtures, like early beta-blockers, often require higher doses, increasing side effects. Single-enantiomer drugs (e.g., levocetirizine) offer enhanced safety but pose synthesis challenges. Ibuprofen’s inactive R-enantiomer (distomer) converts in vivo to the active S-eutomer, justifying racemic use (Brune et al., 1992).
Regulatory Hurdles
Regulators demand enantiomer-specific data, increasing costs. Caldwell (1995) noted disparities in 1990s regulatory approaches, with Europe prioritizing enantiomer data earlier than the U.S. Chiral switching (e.g., esomeprazole from omeprazole) emerged to extend patents and improve profiles (Agranat et al., 2002).
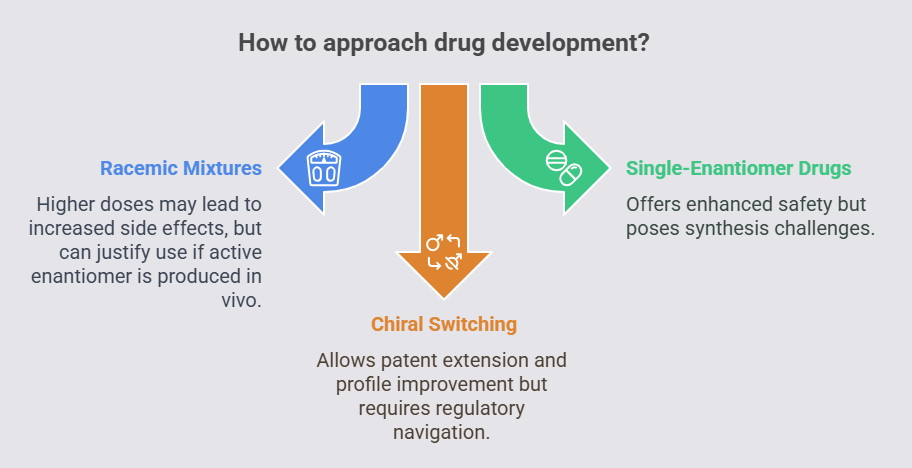
Case Studies
Thalidomide: Despite its history, thalidomide’s R-eutomer treats leprosy and myeloma under strict controls (Franks et al., 2004).
Levodopa: The L-dopa eutomer treats Parkinson’s, while the D-distomer causes granulocytopenia. Modern formulations use pure L-dopa (Wikipedia, “Chiral drugs”).
Beta-Blockers: S-propranolol (eutomer) has 100-fold greater activity than the R-distomer, yet racemic formulations persist due to cost (Tucker, 2000).
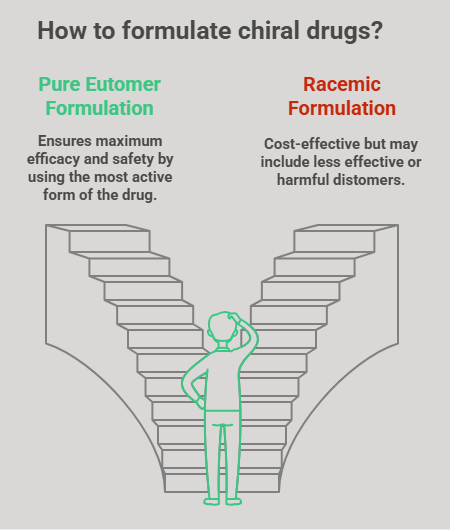
Economic and Ethical Considerations
Single-enantiomer drugs cost 10–30% more but reduce adverse effects (Shah et al., 2000). By the 1990s, 40% of new approvals were enantiopure (Caldwell, 1995). Ethically, rigorous testing prevents tragedies like thalidomide, though affordability debates persist.
Future Directions
Asymmetric Synthesis
Biocatalysis enables Future Directions efficient enantiopure synthesis (Breuer et al., 2004).
Computational Modeling
AI optimizes eudismic ratios by predicting enantiomer-receptor interactions (Yang et al., 2019).
Personalized Medicine
Genetic profiling guides enantiomer selection based on metabolic polymorphisms (Meyer, 2000).
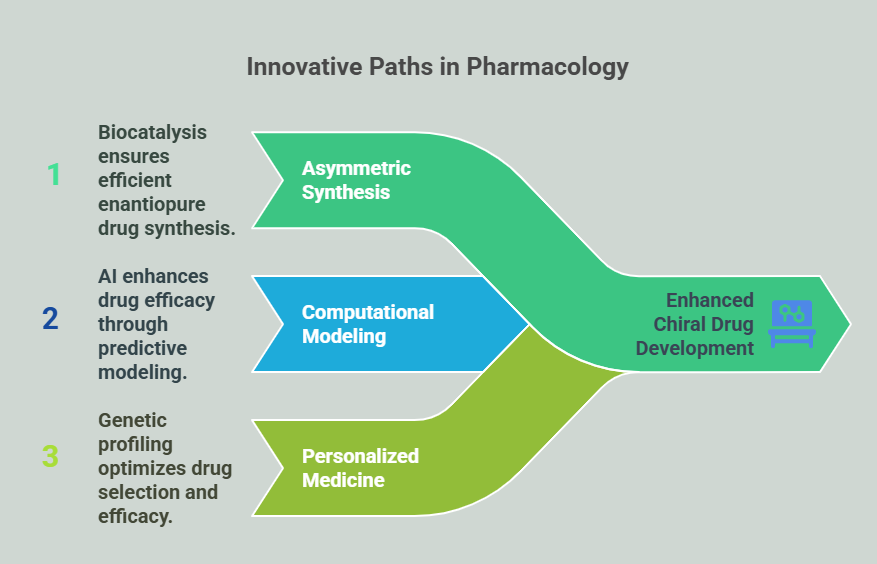
Conclusion
Chiral pharmacology is pivotal in optimizing drug efficacy and safety. Advances in stereochemical understanding, regulatory reforms, and technologies like AI-driven design promise a future of precision medicine. The lessons of thalidomide and the rise of terms like eutomer and eudismic ratio underscore the necessity of embracing chirality in drug development.
References
Ariëns, E. J. (1984). Stereochemistry, a basis for sophisticated nonsense in pharmacokinetics and clinical pharmacology. European Journal of Clinical Pharmacology, 26(6), 663–668.
Blaschke, G., et al. (1980). Chromatographic resolution of racemates. Arzneimittelforschung, 30(2), 258–263.
Caldwell, J. (1995). Chiral pharmacology and the regulation of new drugs. Chemistry and Industry, 6, 176–179.
FDA. (1992). Development of New Stereoisomeric Drugs.
Lehmann, P. A., et al. (1976). Stereoisomerism and biological activity. Progress in Drug Research, 20, 101–142.
Wikipedia contributors. (2023). Chiral drugs. In Wikipedia, The Free Encyclopedia. Retrieved October 15, 2023, from https://en.wikipedia.org/wiki/Chiral_drugs
Yang, Y., et al. (2019). Molecular docking and machine learning for chiral drug design. Journal of Medicinal Chemistry, 62(11), 5262–5281.
Agranat, I., et al. (2002). Putting chirality to work: The strategy of chiral switches. Nature Reviews Drug Discovery, 1(10), 753–768.
Shah, R. R., et al. (2000). The economic impact of chirality in drug development. Drug Discovery Today, 5(8), 323–332.
Kaminsky, L. S., & Zhang, Z. Y. (1997). Human cytochrome P450 metabolism of warfarin. Pharmacology & Therapeutics, 73(1), 67–74.
Tucker, G. T. (2000). Chiral switches. The Lancet, 355(9209), 1085–1087.
Brune, K., et al. (1992). The story of ibuprofen. Agents and Actions Supplements, 36, 9–19.
Further Reading