Asymmetric synthesis is a cornerstone of modern chemistry, enabling the creation of enantiomerically pure compounds essential for pharmaceuticals, materials science, and biotechnology. From early challenges in chiral synthesis to groundbreaking innovations in catalysis, this chapter explores the transformative advancements in asymmetric synthesis, highlighting the scientists, their discoveries, and the profound impact on science and industry.
The Challenge of Selectivity in Synthesis
Synthesis of chiral compounds was once a formidable challenge. Early chemists struggled with racemic mixtures, where equal amounts of enantiomers were produced, leading to inefficiencies in applications requiring specific stereochemical configurations. The ability to selectively synthesize one enantiomer – a process known as asymmetric synthesis – became the “holy grail” of stereochemistry.
For much of the 20th century, methods to achieve enantioselectivity were rudimentary, relying on natural chiral sources or labor-intensive separations. It wasn’t until the mid-20th century that chemists began developing systematic approaches to asymmetric synthesis, laying the groundwork for the revolution in chiral chemistry.
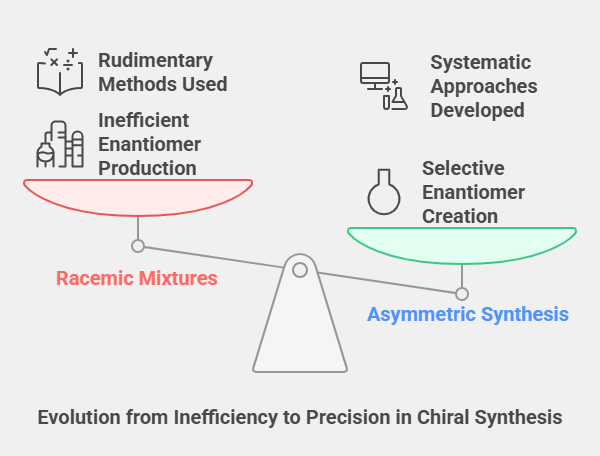
Early Pioneers and Breakthroughs
The journey toward asymmetric synthesis began with incremental breakthroughs:
- Pasteur’s Crystals (1848): Louis Pasteur’s manual separation of tartaric acid crystals marked the first isolation of enantiomers. Though revolutionary, the method’s labor-intensive nature limited its practical applications.
- Chemical Resolutions (1900s): Early chemists used chemical resolutions to separate enantiomers from racemic mixtures. Notable techniques included fractional crystallization and selective reactions with chiral reagents. While effective, these methods lacked the scalability required for industrial applications.
- Chiral Pool Synthesis: Derived from natural sources such as amino acids and sugars, the “chiral pool” served as a reservoir of enantiomerically pure starting materials. Although widely used, this approach was constrained by the availability of suitable chiral precursors.
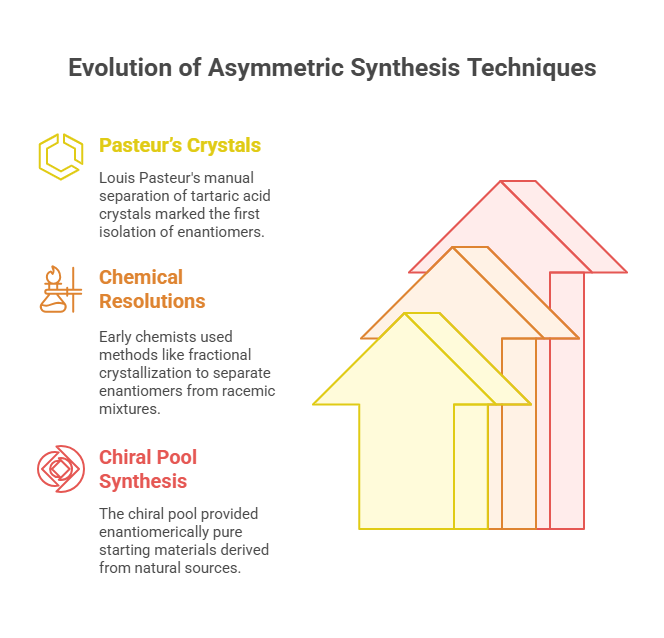
The Catalyst Revolution: Asymmetric Catalysis
The advent of asymmetric catalysis in the mid-20th century transformed chiral synthesis. Catalysts—compounds that accelerate chemical reactions without being consumed – enabled chemists to selectively produce one enantiomer with remarkable efficiency. Key milestones in this revolution include:
Nobel-Winning Innovations in Asymmetric Catalysis
- Proline Catalysis (1970s): In the 1970s, research into amino acid-based catalysts, particularly proline, revealed their potential to drive enantioselective reactions. Proline-mediated aldol reactions became a model system for developing organocatalysis, a field that would later flourish.
- Chiral Metal Complexes: Transition metals such as rhodium, ruthenium, and palladium emerged as powerful catalysts for asymmetric hydrogenation and coupling reactions. William Knowles and Ryōji Noyori’s work on chiral rhodium complexes in the 1970s revolutionized the synthesis of pharmaceuticals, including L-DOPA for Parkinson’s disease.
- Enzymatic Catalysis: Enzymes, nature’s own catalysts, offered unparalleled enantioselectivity. Advances in enzyme engineering and immobilization expanded their use in industrial synthesis, enabling the production of complex chiral molecules.
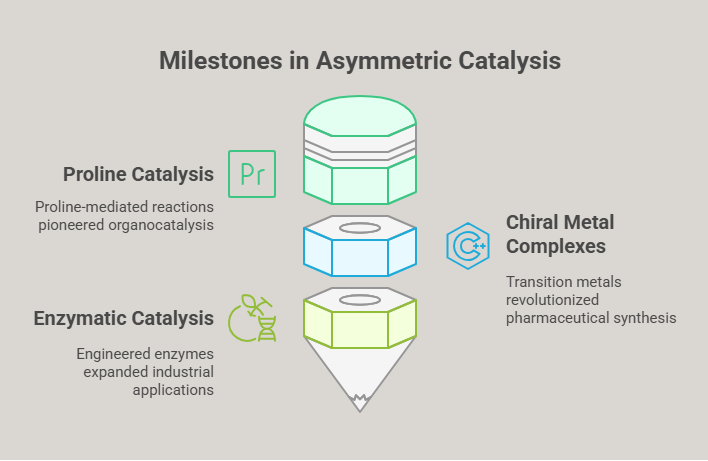
The transformative impact of asymmetric catalysis was recognized with multiple Nobel Prizes:
- William Knowles and Ryōji Noyori (2001): Their pioneering work on asymmetric hydrogenation not only revolutionized pharmaceutical synthesis but also established a blueprint for designing chiral metal catalysts.
- Barry Sharpless (2001): Sharpless’ contributions to asymmetric epoxidation and dihydroxylation provided versatile tools for synthesizing chiral alcohols and epoxides, widely used in medicinal chemistry.
- Benjamin List and David MacMillan (2021): Their independent discovery of organocatalysis, which relies on small organic molecules instead of metals, opened new frontiers in sustainable and environmentally friendly asymmetric synthesis.
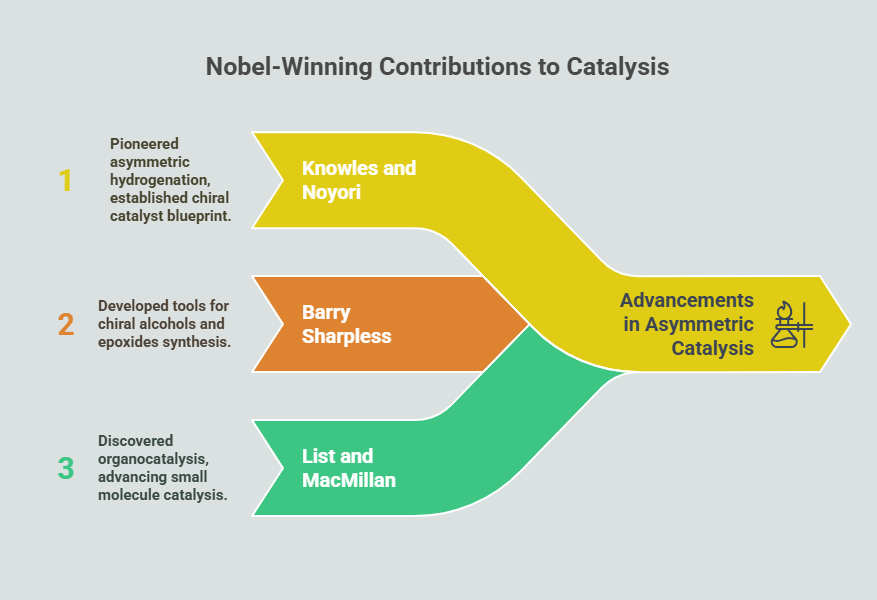
Case Studies in Asymmetric Synthesis
- Asymmetric Hydrogenation: One of the earliest industrial applications of asymmetric catalysis, asymmetric hydrogenation has enabled the large-scale synthesis of chiral pharmaceuticals. For example, the synthesis of L-DOPA – a treatment for Parkinson’s disease – relies on rhodium-based catalysts developed by Knowles.
- Organocatalysis in Drug Synthesis: Organocatalysis has been instrumental in producing complex chiral drugs such as oseltamivir (Tamiflu) and sitagliptin, an antidiabetic medication. By avoiding the use of toxic metals, organocatalysis aligns with the principles of green chemistry.
- Biocatalysis for Green Chemistry: Enzymatic catalysis has found widespread application in the synthesis of antibiotics, statins, and antiviral drugs. The high selectivity and mild reaction conditions of enzymes make them ideal for industrial processes.
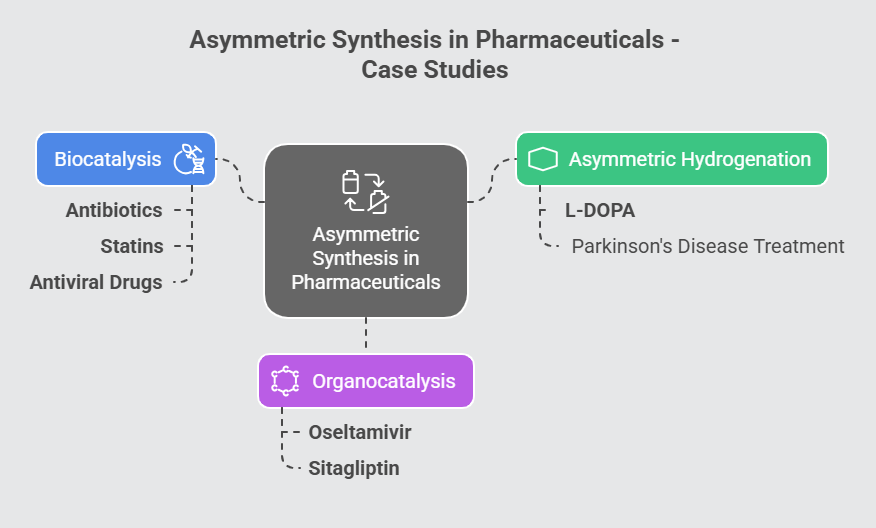
Challenges and Frontiers
While asymmetric synthesis has achieved remarkable success, it continues to face challenges:
- Scalability: Scaling up asymmetric reactions while maintaining enantioselectivity remains a technical hurdle.
- Cost: The development and deployment of chiral catalysts, especially metal-based ones, can be expensive.
- Sustainability: Transitioning to greener methods, such as biocatalysis and organocatalysis, is essential to reduce the environmental footprint of asymmetric synthesis.
- Complexity: Designing catalysts for highly complex molecules, such as natural products and biologics, demands innovative approaches.
The Future of Asymmetric Synthesis
Several emerging trends are shaping the future of asymmetric synthesis:
- AI and Machine Learning: Computational tools are revolutionizing catalyst design by predicting reaction outcomes and optimizing conditions for enantioselective synthesis.
- Photocatalysis and Electrocatalysis: These cutting-edge techniques harness light and electricity to drive enantioselective reactions, offering new possibilities for sustainable synthesis.
- Synthetic Biology: Advances in synthetic biology are enabling the creation of tailor-made enzymes for specific asymmetric transformations, blurring the lines between chemical and biological catalysis.
- Flow Chemistry: Continuous-flow systems are being developed to enhance the efficiency and scalability of asymmetric reactions, making them more practical for industrial applications.
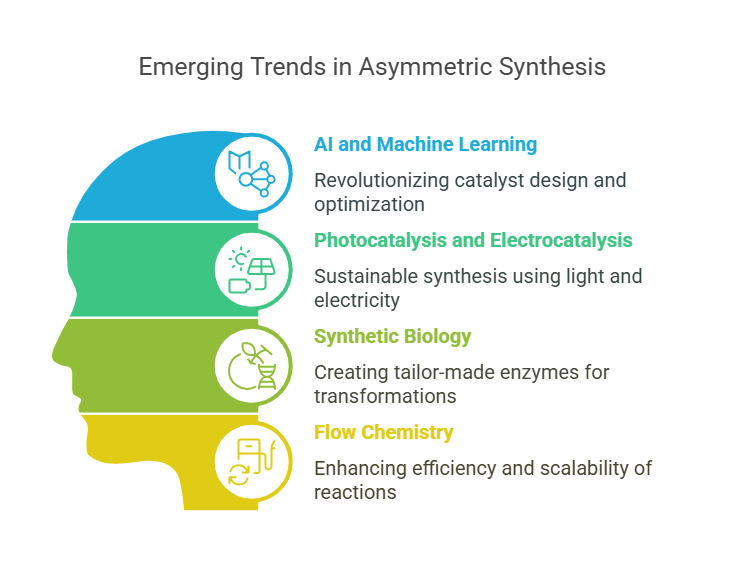
Impact on Industry and Society
The innovations in asymmetric synthesis have far-reaching implications:
- Pharmaceuticals: The ability to produce enantiomerically pure drugs has improved safety and efficacy, transforming the treatment of diseases such as cancer, diabetes, and neurological disorders.
- Materials Science: Asymmetric synthesis is enabling the creation of advanced materials with unique optical, electronic, and mechanical properties.
- Sustainability: By embracing green chemistry principles, asymmetric synthesis is reducing waste and energy consumption, contributing to a more sustainable chemical industry.
Conclusion: Catalysts of Change
The advancements in asymmetric synthesis represent a triumph of human ingenuity and collaboration. From Pasteur’s humble beginnings to the Nobel-winning discoveries of the 21st century, the journey of asymmetric synthesis reflects the power of curiosity and innovation. As we look to the future, the continued exploration of chiral chemistry promises to unlock new possibilities, improving lives and shaping the world around us.
References
Pasteur L (1848). “Recherches sur les propriétés optiques des corps cristallisés.” Comptes rendus de l’Académie des Sciences.
Knowles WS, Noyori R (2001). “Nobel Lectures: Asymmetric Hydrogenation.” Nobelprize.org.Sharpless KB (2001). “Asymmetric Epoxidation and Dihydroxylation.” Journal of the American Chemical Society.
List B, MacMillan DW (2021). “Organocatalysis: The Path to Simplicity and Sustainability.” Angewandte Chemie International Edition.
Sheldon RA (1993). Chirotechnology: Industrial Synthesis of Optically Active Compounds. Marcel Dekker.
Noyori R (2002). “Asymmetric Catalysis in Organic Synthesis.” Angewandte Chemie International Edition.